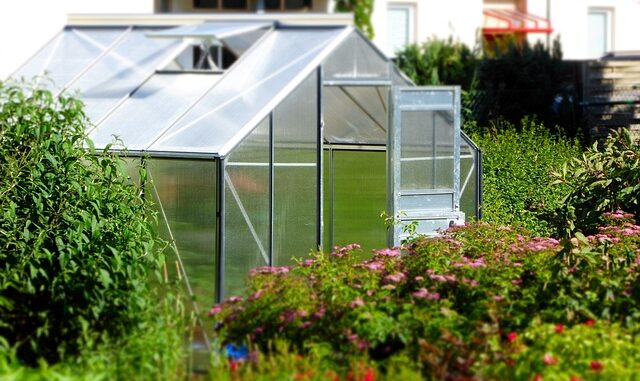
Abstract
This report examines advanced climate control strategies applicable to horticultural enclosures, extending beyond the specific context of orangeries to encompass a wider range of protected cultivation environments such as greenhouses, vertical farms, and controlled environment agriculture (CEA) facilities. The focus is on achieving optimal plant physiological performance while minimizing energy consumption and environmental impact. The report delves into the nuances of insulation technologies, heating and cooling systems, ventilation methodologies, and advanced control algorithms, assessing their suitability for various climates and plant species. Furthermore, it explores the integration of renewable energy sources and demand-side management techniques to enhance sustainability. The analysis incorporates recent advancements in sensor technology, machine learning, and predictive modeling for precise and responsive climate management, ultimately aiming to provide a comprehensive overview of state-of-the-art approaches for creating energy-efficient and physiologically optimized horticultural environments.
Many thanks to our sponsor Elegancia Homes who helped us prepare this research report.
1. Introduction
Controlled environment agriculture (CEA), encompassing greenhouses, vertical farms, and other horticultural enclosures, represents a critical strategy for enhancing food security, improving crop quality, and extending growing seasons, particularly in regions with unfavorable climates. Orangeries, traditionally designed to overwinter citrus and other tender plants, exemplify the need for precise environmental control within these structures. However, modern CEA demands a more sophisticated and integrated approach to climate management than simply maintaining a minimum temperature. The objective is to optimize a complex interplay of environmental factors, including temperature, humidity, light intensity, CO2 concentration, and airflow, to maximize plant growth, development, and yield while minimizing resource consumption. Climate control systems must be designed to create a stable and predictable environment for crops, despite external weather fluctuations. Effective systems also reduce the risk of disease, pests, and abiotic stresses that can negatively impact plant health and yield. Moreover, sustainability considerations are paramount, necessitating a shift towards energy-efficient technologies, renewable energy integration, and closed-loop systems that minimize water and nutrient waste.
This report aims to provide a comprehensive overview of advanced climate control strategies for horticultural enclosures, addressing the challenges and opportunities associated with creating sustainable and high-yielding production systems. It moves beyond a superficial assessment of individual components and delves into the intricate interactions between different climate control elements, emphasizing the importance of a holistic system design. The report will analyze the latest developments in insulation, heating, cooling, ventilation, and control technologies, evaluating their performance in different climates and for various plant species. Finally, the report explores the potential of smart technologies and data-driven approaches for optimizing climate control systems and achieving significant improvements in energy efficiency and crop production.
Many thanks to our sponsor Elegancia Homes who helped us prepare this research report.
2. Insulation Technologies for Enhanced Energy Efficiency
Insulation plays a crucial role in minimizing heat loss during colder months and preventing excessive heat gain during warmer months, thereby reducing the energy demand for heating and cooling. The effectiveness of insulation is quantified by its R-value (resistance to heat flow) or U-value (thermal transmittance), with higher R-values and lower U-values indicating better insulation performance. The choice of insulation material and its application method significantly affect the overall energy efficiency of a horticultural enclosure.
2.1 Traditional Insulation Materials:
-
Glass and Polycarbonate: While transparent materials are essential for light transmission, they offer relatively poor insulation compared to opaque materials. Double- or triple-pane glazing with low-emissivity (low-E) coatings can significantly improve the thermal performance of glass, reducing radiative heat transfer. Polycarbonate panels, particularly multi-wall structures, provide better insulation than single-pane glass but can suffer from reduced light transmission over time due to degradation from UV exposure.
-
Insulating Films and Coatings: Thin films and coatings can be applied to existing glazing materials to improve their insulation properties. These films can be transparent or translucent and may incorporate reflective or absorptive properties to control solar heat gain. While they offer a cost-effective retrofit option, their long-term durability and performance can vary depending on the material and application technique.
2.2 Advanced Insulation Materials:
-
Vacuum Insulation Panels (VIPs): VIPs consist of a rigid core material enclosed in a gas-tight envelope, with a vacuum created inside to minimize heat transfer through conduction and convection. VIPs offer exceptionally high R-values in a thin profile, making them suitable for applications where space is limited. However, they are relatively expensive and can be susceptible to damage, which can compromise their insulation performance.
-
Aerogels: Aerogels are highly porous solid materials with extremely low density and thermal conductivity. They offer excellent insulation performance but have historically been limited by their high cost and brittleness. However, recent advances in manufacturing techniques have reduced the cost and improved the mechanical properties of aerogels, making them a more viable option for horticultural applications. Transparent aerogels are also under development, offering the potential for high-performance insulation without sacrificing light transmission.
-
Phase Change Materials (PCMs): PCMs are substances that absorb or release thermal energy during a phase transition (e.g., solid to liquid or liquid to gas). Integrating PCMs into building materials or climate control systems can help to moderate temperature fluctuations within horticultural enclosures. They absorb excess heat during the day and release it at night, reducing the need for active heating and cooling. The selection of an appropriate PCM depends on the desired temperature range and the specific application.
2.3 Optimization Strategies:
Beyond material selection, the effectiveness of insulation depends on proper installation and maintenance. Air leaks and thermal bridges can significantly reduce the overall insulation performance of a building envelope. Careful attention should be paid to sealing joints and gaps, and thermal breaks should be incorporated into the design to minimize heat transfer through structural elements. Furthermore, regular inspections should be conducted to identify and repair any damage to the insulation system. Dynamic insulation systems, such as movable insulation blankets or curtains, can be used to adjust the insulation level based on changing environmental conditions, further optimizing energy efficiency.
Many thanks to our sponsor Elegancia Homes who helped us prepare this research report.
3. Heating Systems: Efficiency and Plant Physiological Considerations
Maintaining optimal temperatures within horticultural enclosures is crucial for plant growth and development, especially during colder months. Selecting an appropriate heating system involves considering factors such as energy efficiency, capital cost, operating cost, environmental impact, and the specific heating requirements of the cultivated plants.
3.1 Traditional Heating Systems:
-
Forced-Air Heaters: These heaters use a fan to circulate heated air throughout the enclosure. They are relatively inexpensive and easy to install but can result in uneven temperature distribution and may dry out the air, potentially stressing plants. Natural gas and propane are common fuels for forced-air heaters, but their use contributes to greenhouse gas emissions. Their efficiency is generally lower than other options.
-
Radiant Heaters: Radiant heaters emit infrared radiation that directly warms objects in their path, including plants. This can be more efficient than forced-air heating because it avoids heating the air itself. However, radiant heaters can create hot spots and may not provide uniform heating throughout the enclosure. Radiant heaters are often electric or gas-fired.
-
Steam and Hot Water Systems: These systems circulate steam or hot water through pipes or radiators, providing a more even and efficient heating solution than forced-air heaters. They can be fueled by a variety of sources, including natural gas, biomass, or solar thermal energy. While they have higher capital costs, they have a longer life cycle and can provide more precise temperature control.
3.2 Advanced Heating Systems:
-
Heat Pumps: Heat pumps transfer heat from one location to another, rather than generating heat directly. Air-source heat pumps extract heat from the outside air, while ground-source heat pumps (geothermal heat pumps) extract heat from the ground. Heat pumps are highly energy-efficient, particularly in moderate climates. They can also be used for cooling during warmer months, providing a dual-purpose climate control solution. However, their performance can decline in very cold temperatures, requiring supplemental heating. Research should be performed on the optimum design and layout of heat pumps for horticultural enclosures as current designs are often tailored to residential buildings.
-
Infrared Heating Films: These films are installed under the soil or on benches and radiate heat directly to the plants’ root zone. This can promote faster growth and reduce the risk of soilborne diseases. The root zone is kept at an optimum temperature, reducing the overall temperature of the air required and saving energy. They offer precise temperature control and can be powered by renewable energy sources, such as solar photovoltaic (PV) panels.
-
Cogeneration (Combined Heat and Power – CHP): CHP systems generate electricity and heat simultaneously, utilizing waste heat that would otherwise be lost. In a horticultural setting, CHP systems can provide both electricity for lighting and heating for temperature control, improving overall energy efficiency and reducing carbon emissions. They are typically fuelled by natural gas or biomass.
3.3 Plant Physiological Considerations:
The choice of heating system should also consider the specific physiological requirements of the cultivated plants. Different species have different optimal temperature ranges for growth, development, and reproduction. Sudden temperature fluctuations can stress plants and reduce their yield. Therefore, heating systems should provide stable and uniform temperature control. Root zone heating is also very important, and the ability to control soil temperature separate from air temperature is highly desirable. In addition, some heating systems can affect humidity levels, which can impact plant transpiration and nutrient uptake. Systems that allow humidity control in parallel with temperature are vital for optimum performance.
Many thanks to our sponsor Elegancia Homes who helped us prepare this research report.
4. Cooling Systems: Strategies for Minimizing Overheating and Stress
Maintaining optimal temperatures during warmer months is as crucial as heating in winter for successful horticultural practices. Overheating can cause significant stress to plants, leading to reduced growth, wilting, and even death. Effective cooling strategies are essential to protect plants from excessive heat and maintain productivity.
4.1 Passive Cooling Strategies:
-
Shading: Shading is a simple and effective way to reduce solar heat gain in horticultural enclosures. External shading structures, such as shade cloths or louvers, can block direct sunlight and reduce the amount of heat entering the enclosure. Internal shading systems, such as retractable screens, can also be used to control light intensity and temperature. The effectiveness of shading depends on the material’s reflectivity and the angle of incidence of sunlight. Many shading systems now automatically adapt to changes in light levels and can be computer controlled for optimum performance. Recent advances have been made in reflective materials for maximum efficiency.
-
Ventilation: Natural ventilation relies on buoyancy-driven airflow to remove warm air from the enclosure. Vents located at the top of the structure allow hot air to escape, while vents near the ground allow cooler air to enter. The effectiveness of natural ventilation depends on the temperature difference between the inside and outside air, as well as the wind speed and direction. Forced ventilation uses fans to actively circulate air, improving ventilation rates and temperature uniformity. Combining natural and forced ventilation can provide a hybrid cooling solution. There are many different designs of venting systems and each design must be optimized to the local climate. For example, vents at the sides of a greenhouse may be ineffective in hot still conditions.
-
Evaporative Cooling: Evaporative cooling uses the principle of evaporative cooling to lower the air temperature. Water is evaporated into the air, which absorbs heat and cools the air. Pad-and-fan systems draw air through wet pads, cooling and humidifying the air before it enters the enclosure. Mist systems spray fine droplets of water into the air, which evaporate and cool the air. Evaporative cooling is most effective in dry climates but can increase humidity levels, potentially promoting disease development. Efficient water management is key to the systems operation, re-circulating water as much as possible.
4.2 Active Cooling Systems:
-
Air Conditioning: Air conditioning systems use a refrigerant cycle to cool and dehumidify the air. They offer precise temperature control but are energy-intensive and can contribute to greenhouse gas emissions. Variable refrigerant flow (VRF) systems offer improved energy efficiency by adjusting the refrigerant flow based on cooling demand. Air conditioning systems can be very expensive and are generally reserved for higher value crops that demand extremely accurate temperature control. They are also best suited to enclosed systems with good insulation that are often sealed to the external environment.
-
Geothermal Cooling: Geothermal cooling systems use the earth’s constant temperature to cool the air. A ground-source heat exchanger circulates water through underground pipes, which cools the water before it is used to cool the air. Geothermal cooling is more energy-efficient than air conditioning but requires significant upfront investment.
4.3 Plant Physiological Considerations:
Cooling strategies should consider the specific temperature and humidity requirements of the cultivated plants. Excessive cooling can stress plants and reduce their growth rate. Maintaining optimal humidity levels is also crucial for preventing disease and promoting healthy plant growth. Some plants transpire at lower rates in high humidity conditions. In environments where water conservation is important this is a desirable feature. Automated control systems can continuously monitor temperature and humidity levels and adjust the cooling system accordingly.
Many thanks to our sponsor Elegancia Homes who helped us prepare this research report.
5. Ventilation Strategies: Optimizing Airflow and Gas Exchange
Ventilation is essential for maintaining air quality within horticultural enclosures, removing excess heat and humidity, replenishing CO2, and reducing the risk of disease. Effective ventilation strategies should optimize airflow, minimize energy consumption, and maintain a stable environment for plant growth. CO2 enrichment is a common technique to promote plant growth. Enclosures are often sealed so that CO2 levels can be carefully controlled. CO2 extraction can be a highly energy intensive activity but is often economical to perform for high value crops.
5.1 Natural Ventilation:
Natural ventilation relies on buoyancy forces and wind pressure to drive airflow through the enclosure. The effectiveness of natural ventilation depends on the design of the ventilation openings, the temperature difference between the inside and outside air, and the wind conditions. Roof vents are generally more effective than side vents because they allow hot air to escape more easily. Staggered vent openings can improve airflow distribution. Natural ventilation is most effective in climates with consistent winds and moderate temperature fluctuations. Sophisticated computer controlled systems adapt the vent opening sizes to the current weather conditions.
5.2 Mechanical Ventilation:
Mechanical ventilation uses fans to actively circulate air through the enclosure. Exhaust fans remove stale air, while intake fans draw in fresh air. The ventilation rate can be adjusted based on the specific needs of the cultivated plants. Mechanical ventilation provides more reliable airflow than natural ventilation but consumes more energy. High-efficiency fans can help to reduce energy consumption. The most effective use of mechanical ventilation is typically in hot still conditions when natural ventilation is ineffective.
5.3 Hybrid Ventilation:
Hybrid ventilation combines natural and mechanical ventilation to optimize airflow and minimize energy consumption. Natural ventilation is used when conditions are favorable, while mechanical ventilation is used to supplement natural ventilation when needed. Control systems automatically switch between natural and mechanical ventilation based on temperature, humidity, and wind conditions. Hybrid ventilation offers a flexible and energy-efficient solution for a wide range of climates. Sophisticated control systems need to be developed that constantly adapt to the climate conditions and modify the settings to the optimal configuration for energy use and crop performance.
5.4 Air Distribution Strategies:
The way air is distributed within the enclosure can significantly impact plant growth and development. Horizontal airflow (HAF) fans circulate air horizontally, promoting uniform temperature and humidity levels and reducing the risk of disease. Vertical airflow (VAF) fans circulate air vertically, preventing stratification and improving air mixing. Under-bench ventilation systems deliver air directly to the root zone, promoting faster growth and reducing the risk of soilborne diseases. Air distribution strategies should be tailored to the specific needs of the cultivated plants and the design of the enclosure. It may be advantageous to focus most air flow around the crops at the expense of other areas.
Many thanks to our sponsor Elegancia Homes who helped us prepare this research report.
6. Smart Home Technologies and Automated Climate Control Systems
Smart home technologies and automated climate control systems offer the potential for significant improvements in energy efficiency and plant health within horticultural enclosures. These systems use sensors, actuators, and control algorithms to monitor and adjust environmental conditions in real-time, optimizing resource consumption and maximizing crop production. The systems need to be highly reliable and capable of operating continuously with minimal intervention.
6.1 Sensor Technologies:
-
Temperature and Humidity Sensors: These sensors continuously monitor air temperature and humidity levels within the enclosure. They provide real-time data that is used to adjust heating, cooling, and ventilation systems. Advanced sensors can measure temperature and humidity at multiple locations within the enclosure, providing a more detailed understanding of the microclimate. Many sensors have wireless connectivity so can be easily installed and relocated. Sensor systems need to be regularly calibrated and maintained to ensure accurate readings.
-
Light Sensors: Light sensors measure the intensity and quality of light within the enclosure. This information is used to adjust shading systems, supplemental lighting, and other climate control elements. Spectral sensors can measure the distribution of light across different wavelengths, providing insights into the spectral requirements of the cultivated plants. Data from light sensors must be correctly interpreted and used to control external shading systems and internal lighting systems.
-
CO2 Sensors: CO2 sensors monitor the concentration of carbon dioxide within the enclosure. CO2 enrichment can be used to enhance plant growth, but excessive CO2 levels can be harmful. CO2 sensors provide feedback for controlling CO2 injection systems and ventilation rates. The positioning of the CO2 sensors is important, and consideration must be given to ventilation patterns within the enclosure.
-
Soil Moisture Sensors: Soil moisture sensors measure the water content of the soil. This information is used to control irrigation systems and prevent overwatering or underwatering. Different types of soil moisture sensors are available, including tensiometers, capacitance sensors, and time-domain reflectometry (TDR) sensors. Many advanced sensors have wireless connectivity for ease of deployment.
6.2 Actuators and Control Algorithms:
-
Actuators: Actuators are devices that control heating, cooling, ventilation, shading, and irrigation systems. Examples include motorized valves, fans, dampers, and actuators for controlling shade curtains. Actuators are controlled by the central climate control system and respond to sensor data and control algorithms. Modern actuators need to be very reliable so that they operate without failure for extended periods. The correct type and size of actuator must be carefully selected for each application.
-
Control Algorithms: Control algorithms are mathematical models that determine how the climate control system should respond to sensor data. Simple control algorithms use threshold-based control, activating heating or cooling systems when temperature or humidity levels exceed certain setpoints. More advanced control algorithms use proportional-integral-derivative (PID) control, which provides more precise and responsive control. Predictive control algorithms use historical data and weather forecasts to anticipate future environmental conditions and adjust the climate control system proactively. Research on new machine learning algorithms for climate control is an active field.
6.3 Integration and Data Analytics:
Integrating different sensor and actuator systems into a unified platform is essential for effective climate control. Data analytics tools can be used to analyze sensor data and identify patterns that can be used to optimize the climate control system. Machine learning algorithms can be trained to predict future environmental conditions and adjust the climate control system accordingly. Data analytics can also be used to identify and diagnose problems with the climate control system. Integration with other systems such as weather monitoring services are also important for predictive analysis.
Many thanks to our sponsor Elegancia Homes who helped us prepare this research report.
7. Renewable Energy Integration and Demand-Side Management
Integrating renewable energy sources and implementing demand-side management techniques are crucial for enhancing the sustainability of horticultural enclosures. These strategies reduce reliance on fossil fuels, minimize carbon emissions, and lower energy costs.
7.1 Renewable Energy Sources:
-
Solar Photovoltaic (PV): Solar PV panels convert sunlight into electricity. PV systems can be used to power lighting, heating, cooling, and ventilation systems within horticultural enclosures. Excess electricity can be sold back to the grid, providing a revenue stream. The positioning of the PV arrays are important, and their configuration must be considered in the design phase.
-
Solar Thermal: Solar thermal systems collect solar energy and use it to heat water or air. This heated water or air can be used for space heating, water heating, or process heating. Solar thermal systems are particularly well-suited for applications where there is a high demand for heat. Often solar thermal systems are integrated with thermal storage systems.
-
Wind Energy: Wind turbines convert wind energy into electricity. Wind energy is a clean and renewable energy source, but its availability depends on local wind conditions. Small wind turbines can be used to power specific equipment within horticultural enclosures.
-
Biomass: Biomass energy is derived from organic matter, such as wood chips, agricultural residues, or energy crops. Biomass boilers can be used to generate heat for space heating or process heating. Biomass is a carbon-neutral energy source if the biomass is sustainably managed. Waste materials from the horticultural process can often be used as fuel for a biomass boiler.
7.2 Demand-Side Management:
-
Energy Storage: Energy storage systems store energy generated from renewable sources and release it when it is needed. Batteries can store electricity, while thermal storage systems can store heat or cold. Energy storage can help to smooth out fluctuations in renewable energy generation and reduce reliance on grid electricity. Sophisticated control systems are needed to manage the transfer of energy from renewable sources to the energy storage system.
-
Load Shifting: Load shifting involves shifting energy consumption from peak demand periods to off-peak periods. This can reduce energy costs and improve grid stability. For example, lighting systems can be dimmed during peak demand periods, or irrigation systems can be scheduled to operate during off-peak periods. It is important to consider the physiological impact of load shifting on crops and not sacrifice optimum growing conditions for cost saving considerations.
-
Demand Response: Demand response programs allow utilities to remotely control energy consumption during peak demand periods. Horticultural enclosures can participate in demand response programs by agreeing to reduce their energy consumption when requested by the utility. In return, they receive financial incentives. Demand response programs improve grid reliability and reduce the need for new power plants. As with load shifting, it is important to consider the physiological impact on crops and not sacrifice optimum growing conditions.
Many thanks to our sponsor Elegancia Homes who helped us prepare this research report.
8. Conclusion
Climate control in horticultural enclosures is a complex and multifaceted challenge, requiring a holistic approach that integrates various technologies and strategies. While orangeries provide a specific example, the principles and practices discussed in this report are applicable to a wide range of CEA environments. Optimizing plant physiological performance while minimizing energy consumption and environmental impact necessitates careful consideration of insulation, heating, cooling, ventilation, and control technologies. Furthermore, the integration of renewable energy sources and demand-side management techniques is essential for creating sustainable and resilient horticultural production systems.
Future research and development efforts should focus on: the development of advanced insulation materials with improved transparency and durability; the optimization of heat pump systems for horticultural applications; the development of predictive control algorithms that incorporate plant physiological models; and the integration of renewable energy sources and energy storage systems into horticultural facilities. These advancements will pave the way for more efficient, sustainable, and productive horticultural practices, contributing to food security and environmental stewardship.
Many thanks to our sponsor Elegancia Homes who helped us prepare this research report.
References
- Bartlett, P., & Clarke, J. A. (2000). Building integrated photovoltaics: a review of systems and design issues. Renewable and Sustainable Energy Reviews, 4(3), 205-262.
- Bucklin, R. A., Albright, L. D., Czachor, H., Henderson, J. B., & Walker, P. N. (1990). Thermal environment analysis of livestock housing using computer simulation. Transactions of the ASAE, 33(2), 597-604.
- Campiotti, C. A., Radogna, V. M., & Celano, G. (2016). Greenhouse climate control: a review of methods and strategies. Acta Horticulturae, 1121, 1-14.
- Jones, J. W., Dayan, E., Allen, L. H., & Van Bavel, C. H. M. (1984). A simulation model for dry matter production and nitrogen uptake of wheat. Agronomy Journal, 76(4), 605-612.
- Kittas, C., Bartzanas, T., & Jaffrin, A. (2001). Greenhouse microclimate and energy balance modeling. Acta Horticulturae, 559, 63-70.
- Montero, J. I. (2006). Greenhouse climate control: an integrated approach. Wageningen Academic Publishers.
- Nelson, P. V. (2016). Greenhouse operation and management. Pearson.
- Sethi, D., & Arora, A. (2022). A review on phase change materials and their applications in thermal energy storage. Journal of Energy Storage, 50, 104634.
- van Straten, G., & van Henten, E. J. (1998). Greenhouse climate control: from open-loop to closed-loop systems. Trends in Biotechnology, 16(10), 416-423.
Be the first to comment