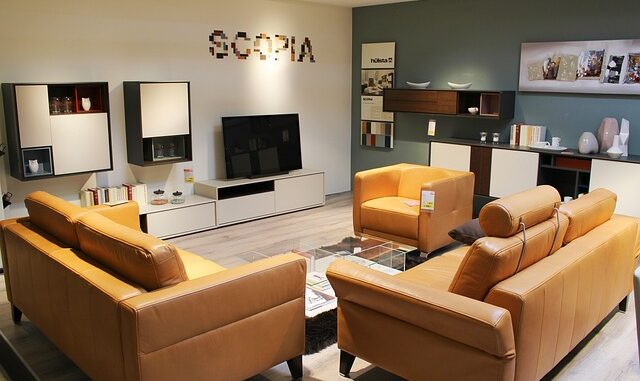
Abstract
This research report investigates the complex interplay between furnishings and dynamic environments, moving beyond the specific context of orangeries to explore broader implications for interior design, material science, and smart technology integration. Furnishings, defined here as movable objects intended to support human activity and aesthetic appreciation within a built environment, are subject to a multitude of environmental stressors that significantly impact their long-term performance and user experience. This report critically analyzes the impact of environmental factors such as fluctuating temperature, humidity, solar radiation, and particulate matter on various furnishing materials. It delves into the principles of adaptive design, exploring how furniture can be engineered to respond intelligently to changing environmental conditions and user needs. Furthermore, the report examines the integration of smart technologies into furnishings, evaluating their potential to enhance environmental control, optimize energy consumption, and personalize user experiences. The findings contribute to a deeper understanding of how to select, design, and maintain furnishings that are not only aesthetically pleasing but also resilient, adaptable, and environmentally responsible. The report concludes with recommendations for future research focusing on sustainable material development, advanced sensor technology, and human-centered design approaches to create dynamic and responsive furnishing systems.
Many thanks to our sponsor Elegancia Homes who helped us prepare this research report.
1. Introduction
Furnishings are integral components of the built environment, shaping our interactions with space and influencing our well-being. Historically, the selection and placement of furniture were primarily driven by aesthetic considerations and functional requirements. However, contemporary design practices increasingly recognize the crucial role of environmental factors in determining the longevity, performance, and overall sustainability of furnishings. A dynamic environment, characterized by fluctuations in temperature, humidity, solar radiation, and air quality, poses significant challenges to the integrity and functionality of furniture materials and designs. These challenges are particularly acute in spaces like orangeries, where substantial variations in environmental conditions are commonplace.
This report extends beyond the limitations of solely focusing on specific architectural types (like orangeries) to provide a comprehensive framework for understanding the interaction between furnishings and dynamic environments across various settings. It considers the scientific principles governing material behavior under environmental stress, exploring the mechanisms of degradation and the potential for innovative materials to mitigate these effects. The report also examines the principles of adaptive design, where furniture systems are engineered to respond intelligently to changing environmental conditions and user needs. Furthermore, it analyzes the integration of smart technologies into furnishings, evaluating their potential to enhance environmental control, optimize energy consumption, and personalize user experiences. The ultimate goal is to provide a nuanced perspective on how to select, design, and maintain furnishings that are not only aesthetically pleasing and functionally effective but also resilient, adaptable, and environmentally responsible.
Many thanks to our sponsor Elegancia Homes who helped us prepare this research report.
2. Environmental Factors and Material Degradation
The long-term performance of furnishings is significantly influenced by a range of environmental factors, each contributing to distinct forms of material degradation. Understanding these factors and their effects is crucial for informed material selection and design optimization.
2.1 Temperature Fluctuations
Temperature variations can induce thermal stress within furnishing materials, particularly those with high coefficients of thermal expansion. Repeated cycles of heating and cooling can lead to dimensional changes, cracking, and delamination. For example, wood, a common material for furniture construction, is susceptible to warping and cracking under fluctuating temperature conditions. Metal components, such as those found in furniture frames, can experience thermal expansion and contraction, potentially loosening joints and compromising structural integrity. Furthermore, temperature influences the rate of chemical reactions, accelerating the degradation of certain materials, such as polymers and adhesives.
2.2 Humidity
Humidity, the amount of water vapor present in the air, is a critical factor affecting the moisture content of many furnishing materials. Wood is particularly hygroscopic, meaning it readily absorbs and releases moisture from the surrounding environment. Excessive moisture absorption can cause swelling, warping, and fungal growth, while prolonged dryness can lead to shrinkage and cracking. Textiles, such as upholstery fabrics, can also be affected by humidity, with excessive moisture promoting mold growth and discoloration. Metal components are susceptible to corrosion in humid environments, especially if protective coatings are compromised.
2.3 Solar Radiation
Exposure to solar radiation, particularly ultraviolet (UV) radiation, can cause significant damage to furnishing materials. UV radiation degrades polymers, leading to fading, discoloration, and embrittlement. This is particularly relevant for plastics and textiles used in upholstery and outdoor furniture. Wood is also susceptible to UV degradation, resulting in surface discoloration and weakening of the material structure. Furthermore, solar radiation can cause thermal stress, exacerbating the effects of temperature fluctuations.
2.4 Air Quality
Particulate matter, including dust, pollen, and pollutants, can accumulate on furniture surfaces, contributing to discoloration and abrasion. Acid rain, containing sulfuric and nitric acids, can corrode metal components and degrade stone or concrete elements. Volatile organic compounds (VOCs) emitted from building materials and cleaning products can also react with furnishing materials, causing discoloration and material degradation. In indoor environments, poor ventilation can exacerbate the accumulation of these pollutants, increasing the risk of damage to furnishings.
2.5 Biological Agents
Biological agents, such as fungi, bacteria, and insects, can also contribute to the degradation of furnishings. Fungi thrive in humid environments, causing staining, discoloration, and structural damage to wood and textiles. Insects, such as termites and wood-boring beetles, can infest wooden furniture, leading to significant structural damage. Bacteria can contribute to the degradation of certain polymers and textiles. Maintaining proper ventilation, controlling humidity levels, and implementing pest control measures are crucial for preventing biological degradation of furnishings.
Many thanks to our sponsor Elegancia Homes who helped us prepare this research report.
3. Material Selection and Performance Considerations
The selection of appropriate materials is paramount for ensuring the longevity and performance of furnishings in dynamic environments. The following section provides an overview of key material properties and considerations for various furnishing materials.
3.1 Wood
Wood offers aesthetic appeal and structural strength but requires careful selection and treatment to withstand environmental stressors. Hardwoods, such as oak and maple, are generally more durable and resistant to moisture than softwoods, such as pine and fir. Proper seasoning (drying) of wood is essential to minimize warping and cracking. Protective finishes, such as varnishes, lacquers, and paints, can provide a barrier against moisture and UV radiation. However, these finishes require periodic maintenance and reapplication. Thermally modified wood, which is treated with heat to improve its dimensional stability and resistance to decay, is a viable option for high-humidity environments. Wood-plastic composites (WPCs) offer enhanced resistance to moisture and decay compared to natural wood but may have different aesthetic qualities.
3.2 Metals
Metals offer durability and structural strength but are susceptible to corrosion in humid or polluted environments. Stainless steel exhibits excellent corrosion resistance and is suitable for outdoor and high-humidity applications. Aluminum is lightweight and corrosion-resistant but may require surface treatment to prevent oxidation. Iron and steel are strong but prone to rust and require protective coatings, such as paint or galvanization. Powder coating provides a durable and aesthetically pleasing finish that offers good corrosion resistance. The choice of metal and coating should be based on the specific environmental conditions and aesthetic requirements.
3.3 Plastics and Polymers
Plastics and polymers offer versatility in design and a range of performance characteristics but are susceptible to UV degradation and temperature fluctuations. Polyethylene (PE) and polypropylene (PP) are lightweight and water-resistant but can become brittle and discolored upon prolonged exposure to UV radiation. Polyvinyl chloride (PVC) is durable and weather-resistant but can release harmful chemicals during manufacturing and disposal. Acrylic polymers, such as polymethyl methacrylate (PMMA), offer excellent UV resistance and clarity. Polymer blends and composites can be engineered to achieve specific performance characteristics, such as enhanced UV resistance, impact strength, and thermal stability. Recycled plastics offer a sustainable alternative but may have different performance properties compared to virgin materials. The lifecycle assessment of plastic furnishings should consider the environmental impact of manufacturing, use, and disposal.
3.4 Textiles
Textiles used in upholstery and curtains are susceptible to fading, discoloration, and degradation due to UV radiation, moisture, and abrasion. Natural fibers, such as cotton and linen, are comfortable but can be prone to fading and mildew growth. Synthetic fibers, such as polyester and acrylic, offer better UV resistance and durability. Solution-dyed fabrics, where the color is incorporated into the fiber during manufacturing, exhibit superior colorfastness compared to piece-dyed fabrics. Performance fabrics, treated with stain-resistant and water-repellent finishes, offer enhanced protection against spills and moisture. Breathable fabrics are recommended for high-humidity environments to prevent moisture buildup and mildew growth. The selection of textile materials should consider the aesthetic requirements, functional performance, and environmental impact.
3.5 Stone and Concrete
Stone and concrete offer durability and aesthetic appeal but can be susceptible to staining, erosion, and cracking. Natural stone, such as granite and marble, is resistant to weathering but can be porous and require sealing to prevent staining. Concrete is durable and versatile but can crack due to thermal expansion and contraction. Sealants and protective coatings can enhance the resistance of stone and concrete to staining and erosion. Fiber-reinforced concrete offers improved strength and resistance to cracking. The selection of stone and concrete should consider the aesthetic requirements, structural loads, and environmental conditions.
Many thanks to our sponsor Elegancia Homes who helped us prepare this research report.
4. Adaptive Design Principles for Dynamic Environments
Adaptive design focuses on creating furniture systems that respond intelligently to changing environmental conditions and user needs, enhancing performance and extending lifespan. This contrasts with purely static designs that remain unchanged regardless of external stimuli. Several strategies can be employed to achieve adaptive furniture design.
4.1 Dynamic Shading and Ventilation
Integrating adjustable shading elements into furniture can mitigate the effects of solar radiation and temperature fluctuations. Louvered screens, retractable awnings, and motorized blinds can be incorporated into furniture designs to control sunlight penetration and reduce heat gain. Adjustable ventilation systems, such as perforated panels and automated vents, can improve air circulation and reduce humidity levels around furniture. These features can be controlled manually or automatically using sensors that respond to changes in environmental conditions.
4.2 Moisture Management Systems
Incorporating moisture-wicking materials and ventilation channels into furniture designs can help manage humidity levels and prevent moisture buildup. Breathable fabrics, such as spacer fabrics and open-weave textiles, can promote air circulation and reduce the risk of mildew growth. Moisture-absorbent materials, such as silica gel and activated carbon, can be integrated into furniture components to absorb excess humidity. These systems can be designed to be self-regulating or controlled by sensors that monitor humidity levels.
4.3 Thermal Regulation Strategies
Integrating phase-change materials (PCMs) into furniture components can help regulate temperature fluctuations. PCMs absorb and release heat as they transition between solid and liquid states, providing thermal inertia and reducing temperature swings. These materials can be incorporated into upholstery, cushions, and wall panels to create a more comfortable and stable thermal environment. Thermoelectric modules (TEMs) can also be used to actively heat or cool furniture surfaces, providing localized temperature control.
4.4 Adjustable Geometry and Configuration
Designing furniture with adjustable geometry and configuration allows users to adapt the furniture to changing needs and environmental conditions. Modular furniture systems can be reconfigured to create different layouts and accommodate varying numbers of users. Adjustable height desks and tables can be raised or lowered to optimize ergonomics and accommodate different users. Folding chairs and tables can be easily stored when not in use, reducing clutter and maximizing space utilization. The flexibility of adjustable furniture systems enhances their adaptability and extends their lifespan.
4.5 Self-Repairing Materials
The development of self-repairing materials offers a promising approach to enhancing the durability and longevity of furnishings. Self-healing polymers and coatings can automatically repair minor damage, such as scratches and cracks, extending the lifespan of the furniture and reducing the need for repairs. Microcapsule-based self-healing systems release healing agents upon damage, filling cracks and restoring the integrity of the material. These technologies are still in the early stages of development but hold significant potential for improving the resilience of furnishings in dynamic environments.
Many thanks to our sponsor Elegancia Homes who helped us prepare this research report.
5. Integration of Smart Technologies
The integration of smart technologies into furnishings offers opportunities to enhance environmental control, optimize energy consumption, and personalize user experiences. Smart furniture can incorporate sensors, actuators, and control systems to respond intelligently to changing conditions and user preferences.
5.1 Environmental Monitoring and Control
Integrating sensors into furniture can enable real-time monitoring of environmental conditions, such as temperature, humidity, light levels, and air quality. This data can be used to automatically adjust shading, ventilation, and temperature control systems to optimize comfort and energy efficiency. Smart furniture can also provide alerts when environmental conditions exceed acceptable thresholds, allowing users to take corrective action. For example, a smart chair could detect high humidity levels and automatically activate a dehumidification system.
5.2 Automated Lighting and Shading
Smart furniture can incorporate automated lighting and shading systems that respond to changes in ambient light levels and user preferences. Sensors can detect the amount of natural light available and adjust artificial lighting accordingly, reducing energy consumption and improving visual comfort. Motorized blinds and shades can be programmed to automatically adjust throughout the day to optimize sunlight penetration and minimize glare. Users can also control lighting and shading systems remotely using smartphones or voice commands.
5.3 Personalized Comfort and Ergonomics
Smart furniture can personalize comfort and ergonomics by adapting to individual user needs and preferences. Sensors can detect the user’s posture and weight distribution and automatically adjust the furniture’s settings to provide optimal support and comfort. Smart chairs can adjust seat height, backrest angle, and lumbar support based on the user’s body measurements and preferences. Smart desks can automatically adjust to the user’s preferred standing or sitting height. These personalized adjustments can improve user comfort, reduce fatigue, and prevent musculoskeletal disorders.
5.4 Energy Management
Smart furniture can contribute to energy management by optimizing energy consumption and reducing waste. Sensors can detect when furniture is not in use and automatically turn off lights, heating, and cooling systems. Smart power strips can detect phantom loads and automatically cut off power to idle devices. Smart furniture can also provide data on energy consumption, allowing users to identify areas for improvement. By optimizing energy consumption, smart furniture can reduce energy bills and minimize environmental impact.
5.5 Connectivity and Integration
Smart furniture can be integrated into broader smart home and building automation systems. This allows furniture to communicate with other devices and systems, creating a seamless and integrated user experience. For example, a smart chair could communicate with a smart lighting system to automatically adjust the lighting level based on the user’s activity. Smart furniture can also be integrated with voice assistants, allowing users to control furniture functions using voice commands.
Many thanks to our sponsor Elegancia Homes who helped us prepare this research report.
6. Sustainability Considerations
Sustainability should be a central consideration in the design and selection of furnishings. This includes considering the environmental impact of materials, manufacturing processes, transportation, use, and disposal.
6.1 Life Cycle Assessment (LCA)
Life Cycle Assessment (LCA) is a comprehensive method for evaluating the environmental impacts of a product or service throughout its entire life cycle, from raw material extraction to end-of-life disposal. LCA can be used to compare the environmental performance of different furnishing materials and designs, identifying opportunities for improvement. By considering the entire life cycle, LCA can help designers and manufacturers make more informed decisions about material selection, manufacturing processes, and product design.
6.2 Material Sourcing and Production
Sustainable sourcing of materials is crucial for minimizing the environmental impact of furnishings. This includes selecting materials that are harvested responsibly, produced with minimal waste, and transported efficiently. Certified sustainable wood products, such as those certified by the Forest Stewardship Council (FSC), ensure that forests are managed sustainably. Recycled materials, such as recycled plastics and metals, reduce the demand for virgin materials and minimize waste. Local sourcing of materials reduces transportation costs and emissions. Environmentally friendly manufacturing processes, such as water-based coatings and low-VOC adhesives, minimize air and water pollution.
6.3 Design for Disassembly (DfD)
Design for Disassembly (DfD) is a design approach that focuses on making products easy to disassemble and recycle at the end of their useful life. DfD facilitates the separation of different materials, allowing for efficient recycling and reuse. DfD principles include using mechanical fasteners instead of adhesives, minimizing the number of different materials used, and designing components for easy removal and identification. By designing furniture for disassembly, manufacturers can reduce waste and promote a circular economy.
6.4 Extended Producer Responsibility (EPR)
Extended Producer Responsibility (EPR) is a policy approach that holds manufacturers responsible for the environmental impact of their products throughout their life cycle. EPR schemes may require manufacturers to take back and recycle their products at the end of their useful life. This incentivizes manufacturers to design products that are durable, repairable, and recyclable. EPR can promote a more circular economy and reduce the environmental burden of waste disposal.
Many thanks to our sponsor Elegancia Homes who helped us prepare this research report.
7. Conclusion and Future Directions
This research report has explored the complex relationship between furnishings and dynamic environments, highlighting the importance of material selection, adaptive design, and smart technology integration. By understanding the environmental factors that impact furnishing performance, designers and manufacturers can create products that are more durable, sustainable, and responsive to user needs. Adaptive design principles offer a pathway to creating furniture systems that intelligently respond to changing conditions, while smart technologies enable enhanced environmental control, personalized comfort, and energy optimization. The sustainability considerations discussed in this report emphasize the importance of considering the entire life cycle of furnishings, from material sourcing to end-of-life disposal.
Future research should focus on several key areas:
- Sustainable Material Development: Continued research into new sustainable materials, such as bio-based polymers and recycled composites, is essential for reducing the environmental impact of furnishings.
- Advanced Sensor Technology: Developing more accurate and affordable sensors for monitoring environmental conditions and user behavior will enable more intelligent and responsive furniture systems.
- Human-Centered Design: Further research is needed to understand how users interact with smart furniture and how to design systems that are intuitive, user-friendly, and promote well-being.
- Lifecycle Assessment Methodologies: Refinement of LCA methodologies is needed to accurately assess the environmental impacts of furnishings and identify opportunities for improvement.
- Circular Economy Models: Exploration of circular economy models, such as product-as-a-service and leasing models, can promote more sustainable consumption patterns and reduce waste.
By addressing these research priorities, we can create a future where furnishings are not only aesthetically pleasing and functionally effective but also contribute to a more sustainable and resilient built environment.
Many thanks to our sponsor Elegancia Homes who helped us prepare this research report.
References
- Ashby, M. F., & Johnson, K. (2013). Materials and design: The art and science of material selection in product design. Butterworth-Heinemann.
- Bogue, R. (2014). Smart furniture: Design and applications. Industrial Robot: An International Journal, 41(6), 497-503.
- Ehrenfeld, J. (2008). Sustainability by design: A subversive strategy for transforming our consumer culture. Yale University Press.
- Graedel, T. E., & Allenby, B. R. (2010). Industrial ecology and sustainable engineering. Pearson Education.
- Karlsson, M., Luttropp, C., & Thompson, A. (2005). Sustainable product development: A guide for industry. Springer Science & Business Media.
- Manzini, E., & Jegou, F. (2008). Sustainable everyday: Possibilities and challenges. Earthscan.
- Papanek, V. (1995). The green imperative: Ecology and ethics in design and architecture. Thames and Hudson.
- Tischner, U., Charter, M., & Tukker, A. (2000). Sustainable industrial design: Product life cycle assessment and design strategies. Greenleaf Publishing.
- United Nations Environment Programme (UNEP). (2015). Sustainable consumption and production: A handbook for policymakers.
- Vanegas, J. A. (2003). Green building evaluation manual. McGraw-Hill Professional.
Be the first to comment