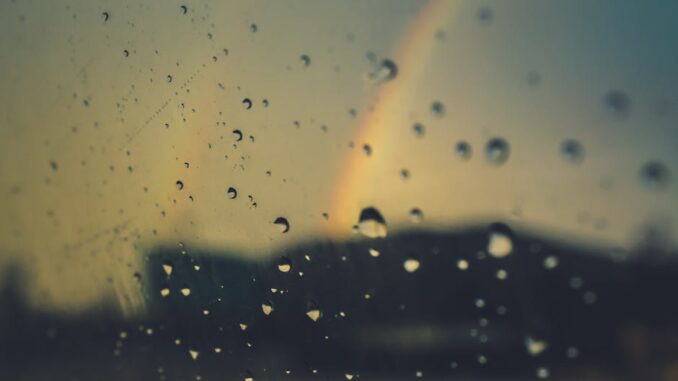
Abstract
Humidity, defined as the concentration of water vapor present in the air, exerts a profound influence across a diverse range of scientific disciplines. Beyond its commonly understood role in meteorology and climate modeling, humidity significantly impacts material science, agricultural practices, industrial processes, and, critically, human health. This research report provides a comprehensive overview of the multifaceted nature of humidity, delving into its measurement techniques, its influence on various physical and biological systems, and the technological approaches used to control and manipulate it. We explore the fundamental principles governing water vapor behavior, examine the effects of humidity on material properties and stability, analyze its role in agricultural productivity and food preservation, and discuss its implications for indoor environmental quality and human well-being. Furthermore, we address the challenges and opportunities associated with humidity control, including the design and optimization of humidification and dehumidification systems, the development of humidity-resistant materials, and the implementation of strategies to mitigate the adverse effects of both excessively high and low humidity levels. This report aims to provide a holistic understanding of humidity, bridging the gap between theoretical concepts and practical applications, and highlighting areas for future research and technological innovation.
Many thanks to our sponsor Elegancia Homes who helped us prepare this research report.
1. Introduction
The presence of water vapor in the atmosphere, quantified as humidity, is a critical environmental parameter with far-reaching consequences. Its influence extends beyond the weather forecast, permeating diverse fields such as material science, agriculture, industrial manufacturing, and human health. Understanding humidity necessitates exploring its fundamental thermodynamic properties, measurement methodologies, and the diverse mechanisms through which it interacts with the environment and biological systems. This report aims to provide a comprehensive overview of these aspects, emphasizing both the challenges and opportunities presented by the control and manipulation of humidity across different domains.
Classical thermodynamics provides the basis for understanding water vapor’s behavior in air. The concentration of water vapor can be described by several metrics, including absolute humidity (mass of water vapor per unit volume of air), specific humidity (mass of water vapor per unit mass of air), mixing ratio (mass of water vapor per unit mass of dry air), and relative humidity (the ratio of the actual water vapor pressure to the saturation vapor pressure at a given temperature). Saturation vapor pressure, a temperature-dependent parameter, defines the maximum amount of water vapor that air can hold at a given temperature. The relationship between these parameters is complex and governed by the Clausius-Clapeyron equation, which describes the exponential increase of saturation vapor pressure with temperature.
Humidity plays a significant role in material science. The presence of water vapor can significantly affect the physical and chemical properties of materials, leading to corrosion, swelling, delamination, and altered mechanical strength. Understanding these effects is crucial in the design and selection of materials for various applications, including construction, electronics, and packaging. In agriculture, humidity influences plant growth, disease development, and post-harvest storage. Controlling humidity in greenhouses and storage facilities is essential for optimizing crop yields and minimizing spoilage.
Indoor air quality is significantly impacted by humidity. Low humidity can lead to dry skin, respiratory irritation, and increased susceptibility to airborne infections. Conversely, high humidity promotes the growth of mold, dust mites, and other allergens, exacerbating respiratory problems and causing structural damage to buildings. Maintaining optimal humidity levels indoors is crucial for ensuring a healthy and comfortable living environment.
The control of humidity is essential across various industrial processes. From pharmaceutical manufacturing to semiconductor fabrication, precise humidity control is necessary to ensure product quality, prevent equipment malfunction, and maintain a safe working environment. Technologies for humidity control include humidifiers, dehumidifiers, air conditioners, and desiccant-based systems. Each technology has its advantages and disadvantages in terms of energy efficiency, cost, and maintenance requirements.
This report will delve into these topics in greater detail, examining the underlying scientific principles, the practical applications, and the technological advancements driving innovation in humidity control.
Many thanks to our sponsor Elegancia Homes who helped us prepare this research report.
2. Measurement Techniques for Humidity
The accurate measurement of humidity is fundamental to various scientific and industrial applications. A diverse array of instruments and techniques have been developed to quantify humidity levels, each with its own advantages, limitations, and suitable application range. This section explores the most prevalent measurement techniques, classifying them based on their operating principles and highlighting their respective strengths and weaknesses.
2.1. Psychrometers
Psychrometers, among the earliest and simplest methods, rely on the principle of evaporative cooling. A psychrometer consists of two thermometers: a dry-bulb thermometer measuring the ambient air temperature and a wet-bulb thermometer with its bulb covered in a wetted wick. As water evaporates from the wick, it cools the wet-bulb thermometer. The difference between the dry-bulb and wet-bulb temperatures, known as the wet-bulb depression, is inversely proportional to the relative humidity. The larger the difference, the lower the humidity, as drier air can absorb more water vapor. Psychrometers are relatively inexpensive and require no external power source. However, they require accurate ventilation to ensure proper evaporation and are sensitive to air velocity. Their accuracy is also limited by the precision of the thermometers and the quality of the wetted wick.
2.2. Hygrometers
Hygrometers represent a broader class of instruments that directly measure humidity using various physical properties of materials that change with humidity. Common types include:
- Hair Hygrometers: These hygrometers utilize the property of human or animal hair to expand and contract in response to changes in humidity. The change in length is mechanically linked to a pointer on a scale indicating relative humidity. While simple and historically significant, hair hygrometers are prone to drift, hysteresis, and require frequent calibration. They are generally less accurate than other types of hygrometers.
- Capacitive Hygrometers: These sensors utilize a capacitor whose dielectric constant changes with humidity. The capacitor is typically constructed with a thin polymer film that absorbs water vapor. As the film absorbs water, its dielectric constant increases, leading to a change in capacitance. This change is measured electronically and correlated to relative humidity. Capacitive hygrometers are compact, relatively inexpensive, and offer good accuracy and response time. They are widely used in HVAC systems, weather stations, and portable humidity meters. However, they can be sensitive to contamination and require periodic calibration.
- Resistive Hygrometers: Similar to capacitive hygrometers, resistive hygrometers utilize a sensor whose electrical resistance changes with humidity. The sensor typically consists of a conductive polymer or a salt-impregnated ceramic substrate. As the sensor absorbs water vapor, its electrical conductivity increases, leading to a decrease in resistance. This change is measured electronically and correlated to relative humidity. Resistive hygrometers are generally less accurate than capacitive hygrometers but offer good long-term stability. They are often used in industrial applications where high accuracy is not critical.
2.3. Dew Point Hygrometers
Dew point hygrometers measure the dew point temperature, which is the temperature at which water vapor in the air will condense into liquid water. The dew point temperature is a direct measure of the absolute humidity of the air. The most common type of dew point hygrometer is the chilled-mirror hygrometer. This instrument consists of a polished mirror that is cooled until condensation forms on its surface. The temperature of the mirror at which condensation occurs is the dew point temperature. Chilled-mirror hygrometers are considered to be among the most accurate humidity measurement devices. They are widely used as reference standards for calibrating other humidity sensors. However, they are relatively expensive and require careful maintenance to ensure accurate operation.
2.4. Spectroscopic Techniques
Spectroscopic techniques offer non-contact and highly sensitive methods for measuring humidity. These techniques rely on the absorption of electromagnetic radiation by water vapor molecules. Common spectroscopic methods include:
- Infrared Absorption Spectroscopy: Water vapor absorbs infrared radiation at specific wavelengths. By measuring the absorption of infrared light at these wavelengths, the concentration of water vapor can be determined. Infrared absorption spectroscopy is widely used in atmospheric monitoring and industrial process control. It offers high sensitivity and selectivity, but it can be affected by the presence of other absorbing gases.
- Tunable Diode Laser Absorption Spectroscopy (TDLAS): TDLAS is a highly sensitive spectroscopic technique that utilizes tunable diode lasers to probe the absorption spectrum of water vapor. By precisely tuning the laser wavelength to a specific absorption line of water vapor, the concentration of water vapor can be measured with high accuracy and precision. TDLAS is widely used in atmospheric research, combustion diagnostics, and leak detection.
2.5. Emerging Technologies
New technologies are continuously emerging for humidity sensing, including microelectromechanical systems (MEMS) sensors, nanomaterial-based sensors, and optical fiber sensors. These technologies offer the potential for smaller size, lower cost, and improved performance compared to traditional humidity sensors. MEMS sensors, for example, can be integrated into portable devices and wireless sensor networks. Nanomaterial-based sensors offer high sensitivity and selectivity due to their large surface area and unique electronic properties. Optical fiber sensors offer immunity to electromagnetic interference and can be used in harsh environments. The development of these emerging technologies is driving innovation in humidity sensing and expanding its applications in various fields.
The choice of humidity measurement technique depends on the specific application requirements, including accuracy, cost, response time, and environmental conditions. Careful consideration should be given to these factors when selecting a humidity sensor for a particular application.
Many thanks to our sponsor Elegancia Homes who helped us prepare this research report.
3. Humidity’s Impact on Material Properties and Stability
The presence of humidity in the surrounding environment significantly influences the physical and chemical properties of materials, leading to a range of effects that can impact their performance, durability, and long-term stability. This section examines these effects in detail, focusing on the mechanisms through which humidity interacts with various material classes and the consequences for their structural integrity and functionality.
3.1. Metals and Corrosion
Corrosion, the degradation of materials due to chemical reactions with their environment, is significantly accelerated by humidity. Water acts as an electrolyte, facilitating the electrochemical reactions that drive corrosion processes. In the presence of oxygen, water promotes the oxidation of metals, leading to the formation of oxides and hydroxides, commonly known as rust. The rate of corrosion increases with increasing humidity and temperature. The presence of pollutants, such as sulfur dioxide and nitrogen oxides, further exacerbates corrosion by forming acidic solutions on the metal surface.
Different metals exhibit varying susceptibility to corrosion. Iron and steel are highly susceptible to rust, while aluminum and stainless steel form protective oxide layers that inhibit further corrosion. However, even these protective layers can be compromised under certain conditions, such as exposure to chlorides or high humidity levels. Strategies to mitigate corrosion include the application of protective coatings, the use of corrosion-resistant alloys, and the control of humidity in enclosed environments.
3.2. Polymers and Plastics
Polymers and plastics are also significantly affected by humidity. Many polymers absorb water vapor, leading to swelling, plasticization, and altered mechanical properties. The extent of water absorption depends on the chemical structure of the polymer and the relative humidity of the environment. Hydrophilic polymers, such as nylon and cellulose, absorb more water than hydrophobic polymers, such as polyethylene and polypropylene.
Water absorption can lead to a decrease in the glass transition temperature (Tg) of the polymer, making it more susceptible to deformation and creep. It can also cause dimensional changes, such as swelling, which can lead to stress cracking and delamination. In addition, water can act as a plasticizer, reducing the stiffness and strength of the polymer. To mitigate these effects, polymers are often modified with additives that reduce water absorption or are used in conjunction with barrier coatings that prevent moisture penetration.
3.3. Composites
Composite materials, consisting of two or more distinct phases, are particularly vulnerable to humidity-induced degradation. Water can penetrate the interface between the different phases, leading to interfacial debonding and loss of mechanical strength. In fiber-reinforced composites, water can diffuse along the fiber-matrix interface, weakening the bond between the fibers and the matrix. This can lead to a reduction in the composite’s stiffness, strength, and fatigue resistance. The use of moisture-resistant resins and surface treatments can help to improve the durability of composites in humid environments.
3.4. Building Materials
Building materials, such as wood, concrete, and brick, are also susceptible to humidity-induced degradation. Wood is hygroscopic, meaning it absorbs and releases moisture from the environment. Changes in moisture content can lead to swelling, shrinkage, and warping. Excessive humidity can also promote the growth of mold and decay fungi, which can weaken the wood structure. Concrete is porous and can absorb water, which can lead to freeze-thaw damage and corrosion of the reinforcing steel. Brick can also absorb water, leading to efflorescence, staining, and cracking. Proper ventilation, drainage, and the use of moisture barriers are essential for protecting building materials from humidity-induced damage.
3.5. Electronic Components
Electronic components are highly sensitive to humidity. Moisture can lead to corrosion of metal conductors, short circuits, and changes in the electrical properties of insulating materials. The presence of moisture can also promote the growth of conductive filaments, known as dendritic growth, which can cause catastrophic failures. Electronic devices are often packaged in sealed enclosures with desiccants to protect them from humidity. Conformal coatings are also applied to printed circuit boards to provide a barrier against moisture and contaminants. In high-humidity environments, it is crucial to use humidity-resistant components and to implement proper moisture control measures.
The influence of humidity on material properties is a complex phenomenon that depends on the material’s composition, structure, and the environmental conditions. Understanding these effects is crucial for selecting appropriate materials for various applications and for implementing strategies to mitigate humidity-induced degradation. Furthermore, the development of new materials with improved humidity resistance is an ongoing area of research and development.
Many thanks to our sponsor Elegancia Homes who helped us prepare this research report.
4. Humidity in Agriculture and Food Preservation
Humidity plays a pivotal role in agricultural productivity, influencing plant growth, disease development, and post-harvest storage. Maintaining optimal humidity levels is essential for maximizing crop yields, minimizing spoilage, and ensuring food safety. This section examines the effects of humidity on various aspects of agriculture and food preservation, highlighting the importance of humidity control in these industries.
4.1. Plant Growth and Development
Humidity affects plant growth and development in several ways. It influences transpiration, the process by which plants lose water through their leaves. High humidity reduces transpiration, which can lead to reduced nutrient uptake and slower growth. Low humidity, on the other hand, can lead to excessive transpiration, causing water stress and wilting. Different plant species have different humidity requirements for optimal growth. Some plants, such as orchids and ferns, thrive in high humidity environments, while others, such as cacti and succulents, prefer low humidity.
Humidity also affects the development of plant diseases. Many fungal and bacterial diseases thrive in high humidity conditions. The presence of free water on plant surfaces promotes spore germination and pathogen infection. Controlling humidity in greenhouses and fields can help to prevent the spread of plant diseases. Ventilation, irrigation management, and the use of fungicides are common strategies for managing humidity in agricultural settings.
4.2. Post-Harvest Storage
Humidity is a critical factor in the post-harvest storage of agricultural products. High humidity promotes the growth of molds, bacteria, and yeasts, which can lead to spoilage and contamination. Low humidity can cause dehydration and shriveling of fruits and vegetables, reducing their quality and marketability. Maintaining optimal humidity levels during storage is essential for extending the shelf life of agricultural products and preventing losses.
Different agricultural products have different humidity requirements for optimal storage. Grains and seeds require low humidity to prevent mold growth and germination. Fruits and vegetables typically require higher humidity to prevent dehydration and maintain their freshness. Controlled atmosphere storage, which involves controlling the temperature, humidity, and gas composition of the storage environment, is used to extend the shelf life of many agricultural products. Refrigeration, ventilation, and the use of packaging materials that control humidity are also important strategies for post-harvest storage.
4.3. Food Processing
Humidity also plays a role in various food processing operations. In drying processes, humidity control is essential for achieving the desired moisture content of the final product. High humidity can slow down the drying process and lead to uneven drying. Low humidity can cause excessive drying and cracking. In baking, humidity affects the texture and crust formation of bread and other baked goods. In fermentation processes, humidity affects the growth and activity of microorganisms. Controlling humidity in food processing facilities is crucial for ensuring product quality and consistency.
4.4. Emerging Technologies
Emerging technologies are being developed to improve humidity control in agriculture and food preservation. These include precision irrigation systems that use sensors to monitor soil moisture and adjust irrigation accordingly, greenhouse climate control systems that use computer algorithms to optimize temperature, humidity, and ventilation, and advanced packaging materials that control humidity and gas exchange. These technologies offer the potential to improve agricultural productivity, reduce food waste, and enhance food safety.
The control of humidity is essential for ensuring the sustainability and efficiency of agricultural production and food preservation. By understanding the effects of humidity on plant growth, disease development, and post-harvest storage, and by implementing appropriate humidity control strategies, we can improve crop yields, reduce food waste, and ensure a safe and secure food supply.
Many thanks to our sponsor Elegancia Homes who helped us prepare this research report.
5. Humidity and Human Health
The impact of humidity on human health is multifaceted, influencing both physiological comfort and the prevalence of various health conditions. This section delves into the complex relationship between humidity and human health, examining the effects of both low and high humidity levels on respiratory function, skin health, and the transmission of infectious diseases.
5.1. Respiratory Health
Low humidity can lead to dryness of the mucous membranes lining the respiratory tract, impairing their ability to trap and remove pathogens and irritants. This can increase susceptibility to respiratory infections, such as the common cold and influenza. Dry air can also exacerbate existing respiratory conditions, such as asthma and bronchitis, by causing inflammation and irritation of the airways. High humidity, on the other hand, can promote the growth of mold, dust mites, and other allergens, which can trigger allergic reactions and asthma attacks. The optimal humidity level for respiratory health is generally considered to be between 40% and 60% relative humidity.
5.2. Skin Health
Low humidity can also lead to dry skin, causing itching, cracking, and irritation. Dry skin is more susceptible to damage and infection. High humidity can exacerbate skin conditions such as eczema and psoriasis by promoting sweating and inflammation. The optimal humidity level for skin health is generally considered to be between 40% and 60% relative humidity. Using moisturizers and avoiding harsh soaps can help to alleviate dry skin in low humidity environments. Proper ventilation and avoiding excessive sweating can help to prevent skin irritation in high humidity environments.
5.3. Infectious Disease Transmission
Humidity plays a significant role in the transmission of infectious diseases, particularly respiratory viruses. Studies have shown that the survival and transmission of influenza viruses are influenced by humidity. Low humidity favors the airborne transmission of influenza viruses, as the virus particles remain suspended in the air for longer periods. High humidity can inactivate influenza viruses by causing them to clump together and settle out of the air. Maintaining optimal humidity levels in indoor environments can help to reduce the spread of respiratory infections.
The exact mechanisms by which humidity affects virus transmission are complex and not fully understood. One hypothesis is that humidity affects the size and density of respiratory droplets, which can influence their ability to travel through the air. Another hypothesis is that humidity affects the stability and infectivity of virus particles. Further research is needed to fully elucidate the relationship between humidity and virus transmission.
5.4. Thermal Comfort
Humidity also affects thermal comfort. High humidity makes it difficult for the body to cool itself through sweating. When sweat evaporates, it removes heat from the body. However, in high humidity environments, the air is already saturated with water vapor, so sweat evaporates more slowly. This can lead to overheating and discomfort. Low humidity can also cause discomfort by drying out the skin and mucous membranes. The optimal humidity level for thermal comfort depends on the temperature and activity level. In general, lower humidity levels are more comfortable at higher temperatures, while higher humidity levels are more comfortable at lower temperatures.
5.5. Indoor Air Quality
Humidity can affect indoor air quality by influencing the growth of mold, dust mites, and other allergens. High humidity promotes the growth of mold and dust mites, which can trigger allergic reactions and asthma attacks. Low humidity can increase the concentration of airborne dust and pollen, which can also trigger allergic reactions. Maintaining optimal humidity levels in indoor environments can help to improve indoor air quality and reduce the risk of allergic reactions and respiratory problems. Proper ventilation, air filtration, and the use of humidifiers and dehumidifiers can help to control humidity and improve indoor air quality.
Maintaining optimal humidity levels is crucial for promoting human health and well-being. By understanding the effects of humidity on respiratory function, skin health, infectious disease transmission, and thermal comfort, we can create healthier and more comfortable indoor environments. This involves implementing strategies to control humidity, such as ventilation, air conditioning, humidification, and dehumidification, as well as promoting awareness of the importance of humidity management for overall health.
Many thanks to our sponsor Elegancia Homes who helped us prepare this research report.
6. Technologies for Humidity Control
Maintaining optimal humidity levels across diverse applications, from residential environments to industrial processes, requires effective and adaptable technologies for both humidification and dehumidification. This section explores the various technologies employed for humidity control, examining their operating principles, advantages, disadvantages, and suitability for different applications.
6.1. Humidification Technologies
Humidification technologies add moisture to the air, increasing the humidity level. Common types of humidifiers include:
- Evaporative Humidifiers: These humidifiers work by evaporating water into the air. A wick or filter absorbs water from a reservoir, and a fan blows air across the wetted surface, causing the water to evaporate. Evaporative humidifiers are relatively inexpensive and self-regulating, as the evaporation rate decreases as the humidity increases. However, they can be noisy, require frequent cleaning to prevent mold growth, and can be less effective in large spaces.
- Ultrasonic Humidifiers: These humidifiers use a vibrating piezoelectric transducer to create a fine mist of water, which is then dispersed into the air. Ultrasonic humidifiers are quiet, energy-efficient, and can produce a large amount of moisture. However, they can produce white dust if hard water is used and can promote the growth of bacteria and mold if not properly maintained.
- Steam Humidifiers: These humidifiers boil water to create steam, which is then released into the air. Steam humidifiers are effective at humidifying large spaces and can kill bacteria and mold in the water. However, they are energy-intensive and can pose a scalding hazard.
- Impeller Humidifiers: These humidifiers use a rotating disk to fling water into the air, creating a fine mist. Impeller humidifiers are relatively inexpensive and easy to maintain. However, they can be noisy and can spread bacteria and mold if not properly cleaned.
The choice of humidifier depends on the size of the space to be humidified, the desired humidity level, the energy efficiency requirements, and the maintenance requirements.
6.2. Dehumidification Technologies
Dehumidification technologies remove moisture from the air, decreasing the humidity level. Common types of dehumidifiers include:
- Refrigerant Dehumidifiers: These dehumidifiers use a refrigerant to cool a coil, causing water vapor to condense on the coil. The condensed water is collected in a reservoir, and the cooled, dehumidified air is then reheated and returned to the room. Refrigerant dehumidifiers are effective at removing large amounts of moisture and are widely used in residential and commercial applications. However, they can be noisy and energy-intensive.
- Desiccant Dehumidifiers: These dehumidifiers use a desiccant material, such as silica gel or zeolite, to absorb moisture from the air. The desiccant material is then heated to release the moisture, which is exhausted to the outside. Desiccant dehumidifiers are effective at removing moisture at low temperatures and humidity levels and are often used in industrial applications. They are generally quieter and more energy-efficient than refrigerant dehumidifiers, but they can be more expensive.
- Thermoelectric Dehumidifiers: These dehumidifiers use a thermoelectric cooler to cool a surface, causing water vapor to condense on the surface. The condensed water is collected in a reservoir, and the cooled, dehumidified air is then reheated and returned to the room. Thermoelectric dehumidifiers are small, quiet, and energy-efficient, but they are less effective at removing large amounts of moisture.
The choice of dehumidifier depends on the size of the space to be dehumidified, the desired humidity level, the temperature, and the energy efficiency requirements.
6.3. Integrated Humidity Control Systems
Integrated humidity control systems combine humidification and dehumidification technologies with sensors and control algorithms to maintain optimal humidity levels in a given environment. These systems can automatically adjust the humidity level based on the temperature, occupancy, and other factors. Integrated humidity control systems are commonly used in HVAC systems, greenhouses, and industrial facilities.
6.4. Emerging Technologies
New technologies are being developed for humidity control, including membrane-based dehumidifiers, liquid desiccant systems, and smart humidity sensors. Membrane-based dehumidifiers use a selective membrane to separate water vapor from the air. Liquid desiccant systems use a liquid desiccant to absorb moisture from the air and then regenerate the desiccant by heating it. Smart humidity sensors provide real-time humidity data and can be integrated into building automation systems to optimize humidity control. These emerging technologies offer the potential for more energy-efficient, cost-effective, and sustainable humidity control solutions.
The selection and implementation of humidity control technologies require careful consideration of the specific application requirements, energy efficiency, cost, and maintenance considerations. As technology advances, new and improved humidity control solutions will continue to emerge, offering the potential for more efficient and sustainable humidity management.
Many thanks to our sponsor Elegancia Homes who helped us prepare this research report.
7. Future Directions and Research Opportunities
The study and control of humidity present numerous avenues for future research and technological innovation. From fundamental investigations into the interaction of water vapor with materials to the development of advanced sensing and control systems, several key areas offer promising opportunities for advancement. This section outlines some of these potential directions, emphasizing the need for interdisciplinary approaches to address the challenges and harness the benefits associated with humidity management.
7.1. Advanced Humidity Sensing
Developing more accurate, reliable, and cost-effective humidity sensors remains a critical area of research. Current sensor technologies have limitations in terms of accuracy, stability, and sensitivity, particularly in harsh environments. Future research should focus on developing new sensing materials and techniques that can overcome these limitations. This includes exploring nanomaterials, optical sensors, and microelectromechanical systems (MEMS) for humidity sensing. Furthermore, the development of wireless and self-powered humidity sensors would enable more widespread and convenient monitoring of humidity in various applications.
7.2. Humidity-Resistant Materials
Developing materials with improved resistance to humidity-induced degradation is essential for enhancing the durability and performance of various products and structures. This includes exploring new polymer formulations, composite materials, and coatings that are less susceptible to water absorption, swelling, and corrosion. Understanding the mechanisms by which humidity interacts with materials at the molecular level is crucial for designing effective humidity-resistant materials. Furthermore, the development of self-healing materials that can repair damage caused by humidity would significantly extend the lifespan of products and structures.
7.3. Energy-Efficient Humidity Control
Improving the energy efficiency of humidification and dehumidification technologies is essential for reducing energy consumption and minimizing environmental impact. Future research should focus on developing more efficient heat exchangers, desiccant materials, and control algorithms for humidity control systems. This includes exploring alternative dehumidification technologies, such as membrane-based dehumidifiers and liquid desiccant systems. Furthermore, optimizing the design and operation of HVAC systems to minimize energy waste is crucial for achieving significant energy savings.
7.4. Impact of Humidity on Human Health
Further research is needed to fully understand the complex relationship between humidity and human health. This includes investigating the effects of humidity on respiratory function, skin health, and the transmission of infectious diseases. Understanding the mechanisms by which humidity affects virus survival and transmission is particularly important for developing effective strategies to prevent the spread of respiratory infections. Furthermore, research is needed to identify the optimal humidity levels for different populations and environments.
7.5. Climate Change and Humidity
Climate change is expected to alter humidity patterns around the world, with some regions becoming wetter and others becoming drier. Understanding these changes and their potential impacts on ecosystems, agriculture, and human health is crucial for developing adaptation strategies. This includes developing climate models that can accurately predict changes in humidity patterns and assessing the vulnerability of different regions to these changes. Furthermore, developing strategies to mitigate the impacts of climate change on humidity-sensitive systems is essential for ensuring a sustainable future.
7.6. Advanced Control Systems
Developing more sophisticated control systems for humidity management is essential for achieving precise and energy-efficient humidity control in various applications. This includes incorporating artificial intelligence and machine learning techniques to optimize humidity control algorithms. Furthermore, integrating humidity control systems with building automation systems and smart grids would enable more intelligent and responsive humidity management.
Addressing these research opportunities will require interdisciplinary collaborations involving materials scientists, engineers, biologists, and climate scientists. By fostering innovation and collaboration, we can unlock the full potential of humidity management for improving human health, protecting the environment, and enhancing the sustainability of various industries.
Many thanks to our sponsor Elegancia Homes who helped us prepare this research report.
References
- ASHRAE. (2019). ASHRAE Handbook – HVAC Applications. American Society of Heating, Refrigerating and Air-Conditioning Engineers.
- Atkins, P. W., & de Paula, J. (2010). Atkins’ Physical Chemistry (9th ed.). Oxford University Press.
- Baraniuk, C. (2020). How humidity affects the spread of coronavirus. BMJ, 369, m1173.
- Derluyn, H., et al. (2013). Critical Assessment of humidity sensors: Overview of sensing mechanisms and applications. Sensors and Actuators A: Physical, 193, 87-108.
- Graedel, T. E., & Frankel, R. S. (1993). Corrosion and Climate. John Wiley & Sons.
- Hsu, H. H., & Tsay, C. B. (2019). Impacts of relative humidity on indoor air quality and human health in air-conditioned environments. Indoor Air, 29(3), 391-405.
- Jones, J. B. (2013). Plant Nutrition and Soil Fertility Manual. CRC press.
- Kaye, J. A. (2008). Chemistry of the Natural Atmosphere (2nd ed.). Elsevier.
- Persily, A., & de Jonge, L. (2017). Measuring and Assessing Ventilation Performance in Buildings. Buildings, 7(3), 68.
- Romney, J. S., et al. (2015). The effect of relative humidity on the infectivity of airborne influenza A virus. PLoS ONE, 10(5), e0123004.
- Wolkoff, P. (2018). Indoor air humidity, air quality, and health—An overview. International Journal of Hygiene and Environmental Health, 221(3), 376-390.
Be the first to comment