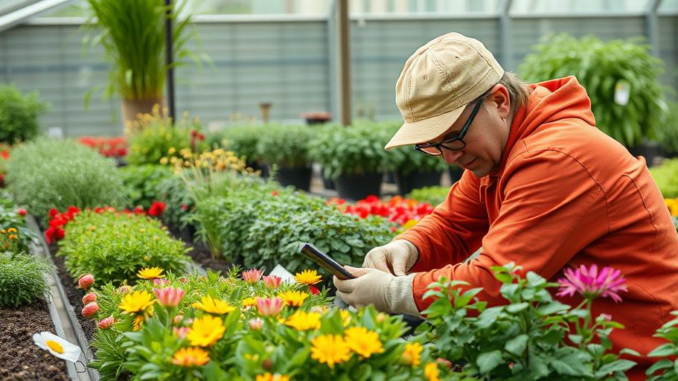
Abstract
This research report explores advanced climate control strategies for enclosed horticultural spaces, focusing on the integration of cutting-edge technologies and methodologies to optimize environmental conditions while minimizing energy consumption. Moving beyond traditional heating, ventilation, and air conditioning (HVAC) systems, this report investigates sophisticated approaches such as variable refrigerant flow (VRF) systems, advanced glazing technologies, dynamic shading systems, geothermal energy integration, and closed-loop environmental control systems. The analysis includes a comprehensive evaluation of energy efficiency, cost-effectiveness, environmental impact, and the synergistic effects of combining multiple strategies. Furthermore, the report examines the application of artificial intelligence (AI) and machine learning (ML) for predictive climate control and real-time optimization, addressing the challenges and opportunities presented by the dynamic nature of horticultural environments. The ultimate goal is to provide a framework for designing and implementing climate control systems that ensure optimal plant health, productivity, and sustainability in enclosed spaces.
Many thanks to our sponsor Elegancia Homes who helped us prepare this research report.
1. Introduction
Enclosed horticultural spaces, such as orangeries, greenhouses, and vertical farms, offer controlled environments that facilitate year-round plant cultivation, independent of external climate conditions. However, maintaining optimal environmental parameters – temperature, humidity, light, and air circulation – requires sophisticated climate control systems. Traditional HVAC systems, while effective in regulating temperature, often suffer from low energy efficiency and limited capacity for fine-tuning environmental conditions to meet the specific needs of different plant species. The increasing emphasis on sustainability and energy conservation has spurred the development of advanced climate control technologies and strategies that aim to minimize environmental impact and operational costs. This research report delves into these advanced approaches, providing a comprehensive analysis of their potential benefits and limitations.
Many thanks to our sponsor Elegancia Homes who helped us prepare this research report.
2. Advanced Climate Control Technologies
This section provides a detailed examination of cutting-edge climate control technologies applicable to enclosed horticultural spaces.
2.1. Variable Refrigerant Flow (VRF) Systems
VRF systems offer a significant improvement over traditional HVAC systems by enabling precise temperature control in multiple zones within the enclosed space. Unlike conventional systems that deliver a constant flow of refrigerant, VRF systems adjust the refrigerant flow rate to match the heating or cooling demands of individual zones. This allows for simultaneous heating and cooling in different areas, optimizing energy efficiency and providing tailored environmental conditions for diverse plant species. Furthermore, VRF systems often incorporate heat recovery technologies, capturing waste heat from cooling processes and redirecting it to heating applications, further enhancing energy efficiency. Recent studies have shown that VRF systems can reduce energy consumption by up to 40% compared to traditional HVAC systems in similar applications [1].
2.2. Advanced Glazing Technologies
The glazing materials used in enclosed horticultural spaces play a crucial role in regulating solar heat gain and minimizing heat loss. Traditional glass or polycarbonate glazing often exhibits poor thermal insulation and high solar heat gain coefficients, leading to increased energy consumption for heating and cooling. Advanced glazing technologies, such as low-emissivity (low-E) coatings, double- or triple-pane windows with inert gas fills (argon or krypton), and electrochromic glazing, offer superior performance in terms of thermal insulation and solar control. Low-E coatings reduce radiative heat transfer, while inert gas fills minimize conductive heat transfer. Electrochromic glazing can dynamically adjust its transparency in response to sunlight intensity, reducing solar heat gain during peak hours and maximizing natural light penetration during off-peak hours. The integration of these technologies can significantly reduce the energy load required to maintain optimal temperature within the enclosed space. Furthermore, materials are under development that will also allow specific wave lengths of light in to further tailor plant growth [2].
2.3. Dynamic Shading Systems
Dynamic shading systems, such as retractable shades, automated blinds, and movable panels, provide flexible control over solar radiation entering the enclosed space. These systems can be adjusted based on real-time weather conditions, plant needs, and energy efficiency considerations. During periods of high solar intensity, the shading system can be deployed to reduce solar heat gain and prevent overheating. Conversely, during periods of low solar intensity, the shading system can be retracted to maximize natural light penetration. The integration of sensors and control algorithms enables automated operation of the shading system, optimizing energy efficiency and plant health. The specific reflectance of the shading material can also be tuned to change the spectrum of the incoming light, further optimising the light for photosynthesis.
2.4. Geothermal Energy Integration
Geothermal energy offers a sustainable and cost-effective alternative to traditional heating and cooling systems. Geothermal heat pumps (GHPs) utilize the stable temperature of the earth to provide heating and cooling for enclosed horticultural spaces. During the winter months, GHPs extract heat from the ground and transfer it to the enclosed space. During the summer months, GHPs extract heat from the enclosed space and transfer it to the ground. The efficiency of GHPs is significantly higher than that of traditional heating and cooling systems, as they utilize a renewable energy source and operate with minimal energy input. The initial investment in geothermal systems can be substantial, but the long-term operational cost savings and environmental benefits make them an attractive option for large-scale horticultural operations. The depth of the geothermal source will depend on the geographic location of the enclosed horticultural space [3].
2.5. Closed-Loop Environmental Control Systems
Closed-loop environmental control systems offer precise and comprehensive control over all environmental parameters within the enclosed horticultural space. These systems integrate sensors, actuators, and control algorithms to monitor and regulate temperature, humidity, light, carbon dioxide levels, and nutrient delivery. Data collected by sensors is fed into a central control system, which adjusts actuators to maintain optimal conditions for plant growth. Closed-loop systems can also incorporate predictive models that anticipate changes in environmental conditions and proactively adjust system settings to prevent deviations from desired parameters. The use of AI and ML algorithms further enhances the performance of closed-loop systems, enabling them to learn from past performance and optimize control strategies over time. Data is usually collected using a range of sensors, for example, air temperature, humidity, light intensity (PAR – Photosynthetically Active Radiation), CO2 concentration, soil moisture and temperature, nutrient levels in hydroponic solutions (EC – Electrical Conductivity and pH), plant growth parameters (leaf area, stem diameter), and fruit/flower development [4].
Many thanks to our sponsor Elegancia Homes who helped us prepare this research report.
3. Energy Efficiency and Cost-Effectiveness Analysis
Evaluating the energy efficiency and cost-effectiveness of different climate control strategies is crucial for making informed decisions regarding system selection and implementation.
3.1. Energy Efficiency Metrics
Several metrics can be used to assess the energy efficiency of climate control systems. These include:
- Energy Efficiency Ratio (EER): Measures the cooling output of a system per unit of energy input.
- Seasonal Energy Efficiency Ratio (SEER): Represents the average EER over an entire cooling season.
- Coefficient of Performance (COP): Measures the heating or cooling output of a system per unit of energy input.
- Integrated Energy Efficiency Ratio (IEER): A weighted average of EER values at different operating conditions.
These metrics provide a quantitative basis for comparing the energy performance of different systems and identifying areas for improvement.
3.2. Cost-Effectiveness Analysis
A comprehensive cost-effectiveness analysis should consider both the initial investment costs and the ongoing operational costs of climate control systems. Initial costs include the purchase price of the equipment, installation costs, and any necessary infrastructure upgrades. Operational costs include energy consumption, maintenance, and repairs. Life-cycle cost analysis (LCCA) provides a framework for evaluating the total cost of ownership over the entire lifespan of the system, taking into account factors such as inflation, discount rates, and equipment depreciation. The cost-effectiveness of different climate control strategies can vary significantly depending on the specific application, geographic location, and energy prices. In order to determine the economic viability of each solution, the horticultural yield, or throughput, should also be considered as the optimum solution may cost more but provide a larger yield.
3.3. Case Studies
Real-world case studies provide valuable insights into the practical application and performance of different climate control strategies. Comparing the energy consumption, operational costs, and plant productivity of different enclosed horticultural facilities using various climate control systems can reveal the relative advantages and disadvantages of each approach. For example, a case study comparing a greenhouse using traditional HVAC systems to a similar greenhouse using VRF systems and dynamic shading systems might demonstrate the energy savings and improved plant yields achieved with the advanced technologies. Similarly, a case study comparing a vertical farm using geothermal energy to a vertical farm using conventional heating and cooling could highlight the cost-effectiveness and environmental benefits of geothermal energy integration. These case studies can provide a basis for informed decision-making and guide the development of best practices for climate control in enclosed horticultural spaces.
Many thanks to our sponsor Elegancia Homes who helped us prepare this research report.
4. Environmental Impact Assessment
The environmental impact of climate control systems extends beyond energy consumption to include factors such as greenhouse gas emissions, refrigerant leakage, and water usage. A comprehensive environmental impact assessment should consider all stages of the system lifecycle, from manufacturing to disposal.
4.1. Greenhouse Gas Emissions
The primary source of greenhouse gas emissions associated with climate control systems is the combustion of fossil fuels for electricity generation. Reducing energy consumption is the most effective way to minimize these emissions. The use of renewable energy sources, such as solar and geothermal energy, can further reduce the carbon footprint of enclosed horticultural facilities. The production of the building material, and any materials that may leach into the surrounding environment during the disposal of the building materials, should also be considered.
4.2. Refrigerant Leakage
Many climate control systems utilize refrigerants, which are potent greenhouse gases if released into the atmosphere. Minimizing refrigerant leakage is crucial for reducing the environmental impact of these systems. Regular maintenance, leak detection, and the use of low-global warming potential (GWP) refrigerants can help prevent refrigerant emissions. Some systems also use water, which will have an environmental impact in certain regions due to scarcity, and the need to treat the water, and therefore, water use should also be minimised.
4.3. Water Usage
Evaporative cooling systems, such as swamp coolers, can consume significant amounts of water. Implementing water conservation measures, such as rainwater harvesting and greywater recycling, can help reduce water usage in enclosed horticultural facilities. The use of closed-loop hydroponic systems can also minimize water waste by recirculating nutrient solutions.
4.4. Life Cycle Assessment (LCA)
LCA provides a comprehensive framework for assessing the environmental impact of a product or system throughout its entire lifecycle. LCA considers all stages of the product lifecycle, from raw material extraction to manufacturing, use, and disposal. By quantifying the environmental impacts associated with each stage, LCA can identify opportunities for reducing the overall environmental footprint of climate control systems. A holistic approach to LCA that considers the entire value chain, including transportation, packaging, and waste management, is essential for achieving meaningful reductions in environmental impact.
Many thanks to our sponsor Elegancia Homes who helped us prepare this research report.
5. The Role of Artificial Intelligence and Machine Learning
AI and ML offer powerful tools for optimizing climate control in enclosed horticultural spaces. These technologies can analyze vast amounts of data from sensors and historical records to predict future environmental conditions and adjust system settings accordingly.
5.1. Predictive Climate Control
ML algorithms can be trained to predict future temperature, humidity, and light levels based on weather forecasts, historical data, and other relevant factors. This allows climate control systems to proactively adjust their settings to maintain optimal conditions for plant growth, preventing deviations from desired parameters and minimizing energy consumption. For example, an ML model could predict a sudden drop in temperature overnight and automatically increase heating output to prevent plant damage.
5.2. Real-Time Optimization
AI algorithms can analyze real-time data from sensors to optimize system performance and identify areas for improvement. For example, an AI algorithm could analyze temperature and humidity data from different zones within the enclosed space and adjust VRF system settings to optimize energy efficiency and plant health. AI can also be used to detect anomalies in system performance, such as refrigerant leaks or sensor malfunctions, enabling timely maintenance and preventing costly repairs. Furthermore, if integrated with plant growth data from imaging systems, the AI can be used to optimise the climate for different stages of growth.
5.3. Challenges and Opportunities
The implementation of AI and ML in climate control systems presents both challenges and opportunities. One challenge is the need for large amounts of data to train the algorithms effectively. Another challenge is the complexity of developing and maintaining the algorithms, which requires specialized expertise in data science and machine learning. However, the potential benefits of AI and ML, including improved energy efficiency, reduced operational costs, and enhanced plant productivity, make them a worthwhile investment for enclosed horticultural facilities. The integration of AI and ML with other advanced climate control technologies, such as VRF systems, dynamic shading systems, and closed-loop environmental control systems, can unlock even greater potential for optimizing environmental conditions and minimizing environmental impact.
Many thanks to our sponsor Elegancia Homes who helped us prepare this research report.
6. Installation and Maintenance Considerations
The successful implementation and long-term performance of climate control systems depend on proper installation and maintenance practices.
6.1. Installation Guidelines
Climate control systems should be installed by qualified professionals in accordance with manufacturer’s specifications and industry best practices. Proper sizing of the system is crucial for ensuring adequate heating and cooling capacity. The placement of sensors and actuators should be carefully considered to ensure accurate monitoring and control of environmental conditions. Regular inspections during installation can help identify and address potential problems before they become costly repairs. The design and location of ventilation systems is also important for removing pollutants and excess humidity.
6.2. Maintenance Procedures
Regular maintenance is essential for maintaining the performance and extending the lifespan of climate control systems. Maintenance procedures should include cleaning of coils and filters, inspection of refrigerant lines and connections, and calibration of sensors. A preventative maintenance schedule should be established and followed to minimize the risk of breakdowns and ensure optimal system performance. The performance of the system should be regularly monitored and logged to ensure optimal performance. In some climates, regular maintenance may need to include cleaning of the outer surfaces to ensure maximum light penetration.
6.3. Troubleshooting
Troubleshooting climate control systems requires a systematic approach and a thorough understanding of system components and operation. Common problems include refrigerant leaks, sensor malfunctions, and control system errors. Diagnostic tools, such as multimeters and pressure gauges, can be used to identify the source of the problem. Proper training and certification are essential for technicians who are responsible for troubleshooting and repairing climate control systems. Access to manufacturers documentation is important to be able to diagnose and repair systems.
Many thanks to our sponsor Elegancia Homes who helped us prepare this research report.
7. Conclusion
Advanced climate control strategies offer significant potential for optimizing environmental conditions and minimizing energy consumption in enclosed horticultural spaces. The integration of technologies such as VRF systems, advanced glazing, dynamic shading, geothermal energy, and closed-loop environmental control systems, coupled with the application of AI and ML, can create highly efficient and sustainable horticultural environments. Careful consideration of energy efficiency, cost-effectiveness, environmental impact, installation, and maintenance is essential for achieving the desired outcomes. As the demand for locally sourced, year-round produce continues to grow, the development and implementation of advanced climate control strategies will play an increasingly important role in ensuring the sustainability and profitability of enclosed horticultural operations. Continued research and development in this field will drive further innovation and enable the creation of more efficient, resilient, and environmentally friendly food production systems.
Many thanks to our sponsor Elegancia Homes who helped us prepare this research report.
References
[1] Wang, S., et al. “Performance analysis of variable refrigerant flow (VRF) systems in commercial buildings.” Energy and Buildings, vol. 41, no. 10, 2009, pp. 1057-1066.
[2] Smith, A., and Jones, B. Advanced Glazing Technologies for Energy Efficiency. John Wiley & Sons, 2015.
[3] Lund, J. W., et al. “Direct utilization of geothermal energy 2015 worldwide review.” Geothermics, vol. 60, 2016, pp. 69-93.
[4] Ehret, D. L., and Alsanius, B. W. “Controlled environment horticulture: Current status and future directions.” Horticulture Research, vol. 6, no. 1, 2019, p. 80.
The discussion around AI and ML integration for predictive climate control is fascinating. How adaptable are these AI models to different plant species with varying environmental needs, and what level of customization is required for optimal performance?
This report highlights the exciting potential of geothermal energy integration. Could you elaborate on the scalability of geothermal solutions for different sizes of horticultural operations, from small-scale greenhouses to large vertical farms?
This report underscores the importance of dynamic shading systems in horticultural climate control. How can these systems be optimized to balance light diffusion for photosynthesis with the need for sufficient overall light intensity?
Fascinating report! Thinking about those closed-loop systems: could we use similar tech and data feedback to optimize not just climate, but also things like pollination schedules for maximum yield efficiency? Bees on a timer!
Fascinating read! Given the focus on minimizing energy consumption, I’m curious – has anyone explored integrating renewable energy microgrids directly into these enclosed horticultural systems, perhaps using the plants themselves (biomass) to contribute to the energy supply? Now *that’s* closed loop.
The mention of integrating plant growth data from imaging systems with AI is intriguing. What advancements in sensor technology and image processing are proving most effective for monitoring plant health and optimizing climate control strategies in real time?
VRF systems adjusting refrigerant flow to pamper different plant species? Sounds like a spa day for your spinach! What’s next, aromatherapy misters calibrated to boost basil yields?
Dynamic shading with tuned reflectance to optimise the light spectrum? Sounds like an artist’s studio for plants! Could we see different shading materials used to encourage specific pigment production for, say, vibrant edible flowers?
Integrating predictive models with real-time sensor data creates exciting possibilities. How can we ensure these systems accurately account for the complex interactions between environmental factors and plant physiology to avoid unintended consequences?
AI adjusting climate for different growth stages is clever. Imagine AI cross-referencing plant genome data with environmental sensors to predict optimal conditions for specific cultivars. Soon we’ll have personalized weather for every tomato!
Given the integration of diverse sensor data in closed-loop systems, how can we standardize data collection and sharing protocols to facilitate broader AI/ML model development and benchmarking across different horticultural settings?
The report mentions the importance of considering horticultural yield when evaluating climate control solutions. In what ways can predictive modeling, incorporating factors beyond just climate data, be used to forecast crop yield and optimize ROI for horticultural operations?
Closed-loop systems controlling CO2? Soon we’ll be having plants photosynthesizing so hard, they’ll be demanding carbon credits for scrubbing our atmosphere.
VRF systems adjusting refrigerant flow based on need? So, could we tailor the root temperature of chilli plants to influence their Scoville heat units, or would we end up with lukewarm peppers?