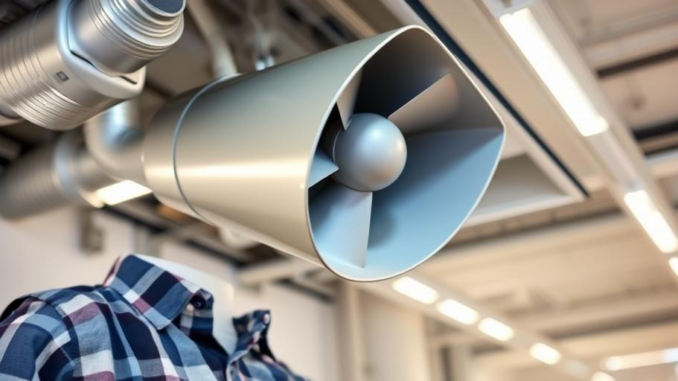
Advanced Ventilation Strategies for Controlled Indoor Environments: Optimizing Air Quality, Thermal Comfort, and Energy Efficiency
Abstract
This research report provides a comprehensive overview of advanced ventilation strategies applicable to controlled indoor environments, extending beyond the basic principles of air exchange to encompass integrated approaches that address air quality, thermal comfort, and energy efficiency. The report delves into the nuances of natural ventilation systems, explores the capabilities of mechanical ventilation with heat recovery (MVHR), evaluates the potential of demand-controlled ventilation (DCV) and displacement ventilation, and examines innovative approaches such as personalized ventilation and hybrid systems. Furthermore, it discusses the importance of computational fluid dynamics (CFD) modeling in ventilation system design and performance evaluation. The report also addresses the challenges associated with ventilation in specific contexts, such as reducing the risk of airborne disease transmission and mitigating the impact of external air pollution. Finally, it identifies promising areas for future research and development in the field of advanced ventilation technologies.
1. Introduction
Ventilation is an essential component of indoor environmental quality, serving to supply fresh air, remove pollutants, and regulate temperature and humidity. Traditional ventilation strategies often rely on constant air volume (CAV) systems, which provide a fixed rate of air exchange regardless of occupancy or indoor air quality demands. However, advancements in building science and engineering have led to the development of more sophisticated ventilation approaches that offer improved performance and energy efficiency. These advanced strategies take into account factors such as occupancy patterns, indoor pollutant sources, climatic conditions, and building envelope characteristics to provide tailored ventilation solutions.
This report aims to provide a comprehensive review of these advanced ventilation strategies, focusing on their principles of operation, performance characteristics, energy efficiency implications, and suitability for various applications. We will explore a range of techniques, including natural ventilation, MVHR, DCV, displacement ventilation, personalized ventilation, and hybrid systems, as well as the role of CFD modeling in optimizing ventilation system design. The report also addresses challenges and future research directions in the field of advanced ventilation.
2. Natural Ventilation Strategies
Natural ventilation leverages natural forces, such as wind pressure and buoyancy-driven thermal currents, to drive air exchange in buildings. This approach offers the potential to reduce reliance on mechanical ventilation systems, leading to significant energy savings and reduced carbon emissions. Effective natural ventilation design requires careful consideration of building orientation, window placement, internal layout, and the prevailing climate. Key natural ventilation strategies include:
- Cross Ventilation: This relies on pressure differences created by wind to draw air through a building from one side to the other. The effectiveness of cross ventilation depends on the size and location of openings, as well as the orientation of the building with respect to the prevailing wind direction. Optimal performance requires strategically placed inlets and outlets that maximize airflow through occupied spaces (Etheridge, 2007).
- Stack Ventilation: This takes advantage of buoyancy forces created by temperature differences between indoor and outdoor air. Warm air rises and exits through high-level openings, while cooler air is drawn in through low-level openings. Stack ventilation is particularly effective in buildings with high ceilings or atria, as it promotes natural convection and vertical air mixing (Liddament, 1996).
- Single-Sided Ventilation: This involves opening windows on only one side of a room or building. While less effective than cross ventilation, single-sided ventilation can still provide some degree of air exchange, particularly in shallow spaces or when combined with other ventilation strategies. The performance of single-sided ventilation is influenced by factors such as window size, orientation, and the presence of internal obstructions (Jiang et al., 2017).
Integrating natural ventilation into building design requires a thorough understanding of local climate conditions and building characteristics. Computational tools such as CFD can be used to simulate airflow patterns and optimize the placement of openings to maximize natural ventilation performance. However, it is important to note that natural ventilation may not be suitable for all climates or building types. In areas with high levels of air pollution or extreme temperatures, mechanical ventilation may be necessary to maintain acceptable indoor air quality and thermal comfort. Furthermore, the need for adequate security and acoustic isolation may also limit the applicability of natural ventilation.
3. Mechanical Ventilation with Heat Recovery (MVHR)
Mechanical Ventilation with Heat Recovery (MVHR) systems provide a controlled and energy-efficient means of supplying fresh air to buildings while minimizing heat loss. MVHR systems use a heat exchanger to transfer heat between incoming fresh air and outgoing exhaust air, thereby pre-heating or pre-cooling the incoming air and reducing the energy required to maintain comfortable indoor temperatures. MVHR systems are particularly well-suited for airtight buildings, where natural ventilation is limited and mechanical ventilation is necessary to ensure adequate air exchange.
The key components of an MVHR system include:
- Supply Fan: This fan draws fresh air from outside and delivers it to the building’s ventilation ducts.
- Exhaust Fan: This fan extracts stale air from the building and expels it outside.
- Heat Exchanger: This component transfers heat between the incoming and outgoing air streams without allowing them to mix. Common types of heat exchangers used in MVHR systems include plate heat exchangers, rotary heat exchangers, and heat pipes.
- Filters: Filters are used to remove pollutants from both the incoming and outgoing air streams, improving indoor air quality and protecting the heat exchanger from fouling.
MVHR systems offer several advantages over traditional mechanical ventilation systems:
- Energy Efficiency: By recovering heat from exhaust air, MVHR systems can significantly reduce heating and cooling energy consumption, leading to lower energy bills and reduced carbon emissions.
- Improved Indoor Air Quality: MVHR systems provide a constant supply of fresh, filtered air, removing pollutants such as dust, pollen, and volatile organic compounds (VOCs). This can improve indoor air quality and reduce the risk of respiratory problems.
- Reduced Humidity: MVHR systems can help to control indoor humidity levels by removing excess moisture from the air. This can prevent the growth of mold and mildew and improve occupant comfort.
However, MVHR systems also have some disadvantages:
- Cost: MVHR systems are typically more expensive to install than traditional mechanical ventilation systems.
- Maintenance: MVHR systems require regular maintenance, including filter replacement and cleaning of the heat exchanger.
- Noise: MVHR systems can generate some noise, although this can be minimized through careful design and installation.
4. Demand-Controlled Ventilation (DCV)
Demand-Controlled Ventilation (DCV) is a ventilation strategy that adjusts the ventilation rate based on real-time occupancy levels or indoor air quality parameters. This approach ensures that ventilation is provided only when and where it is needed, reducing energy consumption and improving indoor air quality. DCV systems typically use sensors to monitor occupancy, carbon dioxide (CO2) levels, or other pollutants, and then adjust the ventilation rate accordingly.
Key parameters for DCV include:
- Occupancy Sensing: DCV systems can use occupancy sensors to detect the presence of people in a room or building. When occupancy is low, the ventilation rate is reduced, and when occupancy is high, the ventilation rate is increased. Common types of occupancy sensors include infrared sensors, ultrasonic sensors, and microwave sensors.
- CO2 Sensing: CO2 is a byproduct of human respiration and is often used as an indicator of occupancy levels. DCV systems can use CO2 sensors to monitor CO2 concentrations in the air and adjust the ventilation rate accordingly. When CO2 levels are high, the ventilation rate is increased, and when CO2 levels are low, the ventilation rate is reduced (Persily, 1997).
- VOC Sensing: Volatile organic compounds (VOCs) are emitted from a variety of sources, including building materials, furniture, and cleaning products. DCV systems can use VOC sensors to monitor VOC concentrations in the air and adjust the ventilation rate accordingly. When VOC levels are high, the ventilation rate is increased, and when VOC levels are low, the ventilation rate is reduced.
DCV systems offer several advantages over CAV systems:
- Energy Savings: DCV systems can significantly reduce energy consumption by providing ventilation only when and where it is needed. Studies have shown that DCV can reduce ventilation energy consumption by up to 50% in some applications (Emmerich et al., 2000).
- Improved Indoor Air Quality: DCV systems can improve indoor air quality by providing ventilation in response to real-time pollutant levels. This ensures that pollutants are removed from the air as quickly as possible, reducing the risk of health problems.
- Cost Savings: While DCV systems may have a higher initial cost than CAV systems, the energy savings can often offset the higher cost over the life of the system.
However, DCV systems also have some disadvantages:
- Complexity: DCV systems are more complex than CAV systems and require more sophisticated controls and sensors.
- Cost: The initial cost of DCV systems can be higher than that of CAV systems.
- Maintenance: DCV systems require regular maintenance to ensure that the sensors are functioning properly and that the controls are calibrated correctly.
5. Displacement Ventilation
Displacement ventilation is a ventilation strategy that supplies air at a low velocity from floor-level diffusers and extracts air at a high level near the ceiling. The principle behind displacement ventilation is that warm air rises due to buoyancy, carrying pollutants and contaminants with it. The low-velocity supply air displaces the warm, contaminated air upward, creating a stratified air environment with cleaner air near the floor level and more polluted air near the ceiling. Displacement ventilation is well-suited for spaces with high ceilings and low occupancy density, such as theaters, auditoriums, and industrial facilities.
Key features of displacement ventilation systems include:
- Low-Velocity Supply Air: Air is supplied at a low velocity (typically less than 0.5 m/s) to minimize mixing and maintain stratification.
- Floor-Level Diffusers: Air is supplied through diffusers located near the floor, allowing it to rise naturally due to buoyancy.
- High-Level Exhaust: Air is extracted at a high level near the ceiling, removing the warm, contaminated air that has risen to the top of the space.
Displacement ventilation offers several advantages over mixing ventilation systems:
- Improved Air Quality: Displacement ventilation can improve air quality by removing pollutants and contaminants from the occupied zone more effectively than mixing ventilation systems. This can reduce the risk of respiratory problems and improve occupant comfort.
- Energy Savings: Displacement ventilation can reduce energy consumption by supplying air at a lower temperature than mixing ventilation systems. This reduces the cooling load on the HVAC system.
- Reduced Drafts: Displacement ventilation systems typically produce fewer drafts than mixing ventilation systems, improving occupant comfort.
However, displacement ventilation also has some limitations:
- Sensitivity to Heat Sources: Displacement ventilation is sensitive to heat sources within the space. High heat loads can disrupt the stratification and reduce the effectiveness of the system.
- Ceiling Height Requirements: Displacement ventilation requires relatively high ceilings to function effectively. In spaces with low ceilings, the stratification may not be well-established, reducing the benefits of the system.
- Occupancy Density Limitations: Displacement ventilation is best suited for spaces with low occupancy density. In spaces with high occupancy density, the air may become too mixed, reducing the effectiveness of the system.
6. Personalized Ventilation
Personalized ventilation (PV) is a ventilation strategy that provides individual control over the air supply to each occupant. This approach allows occupants to adjust the airflow rate, direction, and temperature to suit their individual preferences and needs. PV systems can improve occupant comfort, reduce the risk of airborne disease transmission, and increase productivity. PV systems typically consist of a central air handling unit that supplies conditioned air to individual workstations or seating areas, each equipped with an adjustable diffuser or nozzle.
Key components of PV systems include:
- Central Air Handling Unit: This unit provides conditioned air (filtered, heated, or cooled) to the individual workstations.
- Individual Diffusers or Nozzles: Each workstation is equipped with an adjustable diffuser or nozzle that allows occupants to control the airflow rate, direction, and temperature.
- Sensors: Sensors may be used to monitor occupancy, temperature, and air quality in the individual workstations.
- Controls: Controls allow occupants to adjust the airflow rate, direction, and temperature of the air supplied to their workstation.
PV systems offer several advantages over traditional ventilation systems:
- Improved Occupant Comfort: PV systems allow occupants to customize their air environment, improving comfort and reducing complaints about temperature and drafts.
- Reduced Risk of Airborne Disease Transmission: PV systems can reduce the risk of airborne disease transmission by delivering clean air directly to each occupant and removing contaminated air from the breathing zone (Melikov, 2004).
- Increased Productivity: Studies have shown that PV systems can increase productivity by improving occupant comfort and reducing distractions.
However, PV systems also have some limitations:
- Cost: PV systems can be more expensive to install than traditional ventilation systems.
- Complexity: PV systems are more complex than traditional ventilation systems and require more sophisticated controls and sensors.
- Maintenance: PV systems require regular maintenance to ensure that the diffusers or nozzles are functioning properly and that the controls are calibrated correctly.
7. Hybrid Ventilation Systems
Hybrid ventilation systems combine natural ventilation with mechanical ventilation to provide a flexible and energy-efficient ventilation solution. These systems typically use natural ventilation when outdoor conditions are favorable and switch to mechanical ventilation when outdoor conditions are unfavorable or when natural ventilation is insufficient to meet indoor air quality requirements. Hybrid ventilation systems can offer the benefits of both natural and mechanical ventilation, while minimizing the drawbacks of each approach.
Common types of hybrid ventilation systems include:
- Natural Ventilation with Mechanical Exhaust: This system uses natural ventilation as the primary means of air supply and mechanical exhaust to remove stale air from the building. This approach is particularly well-suited for buildings with good access to natural ventilation, but where mechanical exhaust is needed to ensure adequate air exchange.
- Mechanical Ventilation with Natural Supply: This system uses mechanical ventilation to supply fresh air to the building and natural ventilation to exhaust stale air. This approach is suitable for buildings where natural ventilation is limited, but where natural exhaust can provide some degree of air exchange.
- Mixed-Mode Ventilation: This system uses both natural and mechanical ventilation simultaneously. The system may switch between natural and mechanical ventilation based on outdoor conditions, occupancy levels, or other factors. Mixed-mode ventilation can offer the greatest degree of flexibility and energy efficiency.
Hybrid ventilation systems require sophisticated controls to manage the transition between natural and mechanical ventilation. These controls must take into account factors such as outdoor temperature, humidity, wind speed, occupancy levels, and indoor air quality parameters. The control system should be designed to maximize the use of natural ventilation while ensuring that indoor air quality and thermal comfort are maintained.
8. Computational Fluid Dynamics (CFD) Modeling
Computational Fluid Dynamics (CFD) modeling is a powerful tool for analyzing and optimizing ventilation system performance. CFD simulations can be used to predict airflow patterns, temperature distribution, and pollutant concentrations within a building, allowing engineers to evaluate the effectiveness of different ventilation strategies and identify potential problems before construction. CFD modeling can be particularly useful for designing complex ventilation systems, such as displacement ventilation or personalized ventilation, where traditional design methods may be inadequate. Furthermore, CFD simulations are increasingly being used to evaluate ventilation strategies during the design phase in order to minimize the risk of airborne transmission of infectious disease.
CFD simulations involve creating a mathematical model of the building and its ventilation system, and then solving the governing equations of fluid flow and heat transfer to predict the airflow patterns and temperature distribution. The accuracy of the CFD simulation depends on the quality of the model and the accuracy of the input data. CFD simulations require significant computational resources and expertise, but the results can provide valuable insights into ventilation system performance.
CFD modeling can be used to:
- Optimize the Placement of Air Inlets and Outlets: CFD simulations can be used to determine the optimal placement of air inlets and outlets to maximize airflow through occupied spaces and minimize dead zones.
- Evaluate the Effectiveness of Different Ventilation Strategies: CFD simulations can be used to compare the performance of different ventilation strategies, such as displacement ventilation, mixing ventilation, and personalized ventilation.
- Predict Temperature Distribution: CFD simulations can be used to predict temperature distribution within a building, allowing engineers to identify areas that may be too hot or too cold.
- Analyze Pollutant Dispersion: CFD simulations can be used to analyze the dispersion of pollutants within a building, allowing engineers to evaluate the effectiveness of ventilation systems in removing pollutants from the air.
9. Ventilation and Airborne Disease Transmission
The COVID-19 pandemic has highlighted the importance of ventilation in reducing the risk of airborne disease transmission. Ventilation can dilute and remove airborne pathogens, reducing the concentration of infectious particles in the air and lowering the risk of infection. The effectiveness of ventilation in reducing airborne disease transmission depends on the ventilation rate, the air distribution patterns, and the characteristics of the pathogens. Ventilation can be combined with other measures, such as air filtration and disinfection, to further reduce the risk of airborne disease transmission.
Strategies for improving ventilation to reduce airborne disease transmission include:
- Increasing Ventilation Rates: Increasing the ventilation rate can dilute and remove airborne pathogens more effectively. This can be achieved by opening windows, increasing the airflow rate in mechanical ventilation systems, or using portable air purifiers.
- Improving Air Distribution: Improving air distribution can ensure that clean air is delivered to occupied spaces and that contaminated air is removed effectively. This can be achieved by adjusting the placement of air inlets and outlets or using displacement ventilation systems.
- Using Air Filtration: Air filtration can remove airborne pathogens from the air. High-efficiency particulate air (HEPA) filters are particularly effective at removing small particles, including viruses and bacteria. Portable air purifiers with HEPA filters can be used to supplement ventilation in areas with high occupancy or poor ventilation (ASHRAE, 2020).
- Using Ultraviolet Germicidal Irradiation (UVGI): UVGI can kill or inactivate airborne pathogens. UVGI systems use ultraviolet light to disinfect the air. UVGI can be used in combination with ventilation to further reduce the risk of airborne disease transmission.
10. Challenges and Future Directions
Despite the advancements in ventilation technology, several challenges remain. These challenges include:
- Cost: Advanced ventilation systems can be more expensive to install and maintain than traditional ventilation systems. This can be a barrier to adoption, particularly in developing countries.
- Complexity: Advanced ventilation systems are more complex than traditional ventilation systems and require more sophisticated controls and sensors. This can make them more difficult to design, install, and operate.
- Integration: Integrating advanced ventilation systems with other building systems, such as heating, cooling, and lighting, can be challenging. This requires a holistic approach to building design and operation.
- Occupant Behavior: Occupant behavior can significantly impact the performance of ventilation systems. For example, occupants may close windows or adjust thermostats in ways that compromise the effectiveness of the system.
Future research and development in the field of advanced ventilation should focus on addressing these challenges. Promising areas for future research include:
- Developing low-cost, high-performance ventilation systems: Research is needed to develop ventilation systems that are affordable and effective, particularly for use in developing countries.
- Developing simpler, more robust controls: Research is needed to develop controls that are easier to use and less prone to failure. Artificial intelligence and machine learning could play a role in developing more intelligent and adaptive control systems.
- Improving integration with other building systems: Research is needed to develop integrated building management systems that can optimize the performance of all building systems, including ventilation, heating, cooling, and lighting. Building information modeling (BIM) can be a valuable tool for facilitating this integration.
- Developing occupant-centric ventilation systems: Research is needed to develop ventilation systems that are more responsive to occupant needs and preferences. This could involve using sensors to monitor occupant activity and adjust ventilation rates accordingly, or developing personalized ventilation systems that allow occupants to control their own air supply.
- Investigating the impact of ventilation on health and well-being: More research is needed to understand the impact of ventilation on human health and well-being. This could involve studying the effects of different ventilation strategies on respiratory health, cognitive performance, and sleep quality.
11. Conclusion
Advanced ventilation strategies offer significant potential to improve indoor air quality, thermal comfort, and energy efficiency in buildings. By carefully considering the principles of natural ventilation, MVHR, DCV, displacement ventilation, personalized ventilation, and hybrid systems, and by utilizing CFD modeling to optimize system design, engineers can create ventilation solutions that are tailored to the specific needs of each building and its occupants. While challenges remain, ongoing research and development efforts are paving the way for more cost-effective, reliable, and occupant-centric ventilation systems that can contribute to healthier and more sustainable built environments. The increasing awareness of the importance of ventilation in preventing airborne disease transmission further reinforces the need for continued innovation in this field.
References
- ASHRAE. (2020). ASHRAE Position Document on Airborne Infectious Diseases. Atlanta, GA: ASHRAE.
- Emmerich, S. J., Persily, A. K., & Nabinger, S. J. (2000). Measured energy savings and demand control ventilation performance in a new office building. ASHRAE Transactions, 106(2), 457-471.
- Etheridge, D. (2007). Natural Ventilation of Buildings: Theory, Measurement and Design. John Wiley & Sons.
- Jiang, Y., Haghighat, F., & Lee, C. S. (2017). A review on parameters influencing single-sided natural ventilation and available modeling techniques. Renewable and Sustainable Energy Reviews, 76, 1319-1336.
- Liddament, M. W. (1996). A guide to energy efficient ventilation. AIVC Guide 17.
- Melikov, A. K. (2004). Personalized ventilation. Indoor Air, 14(s7), 157-167.
- Persily, A. (1997). Carbon dioxide as an indicator of ventilation performance. ASHRAE Journal, 39(10), 32-39.
Be the first to comment