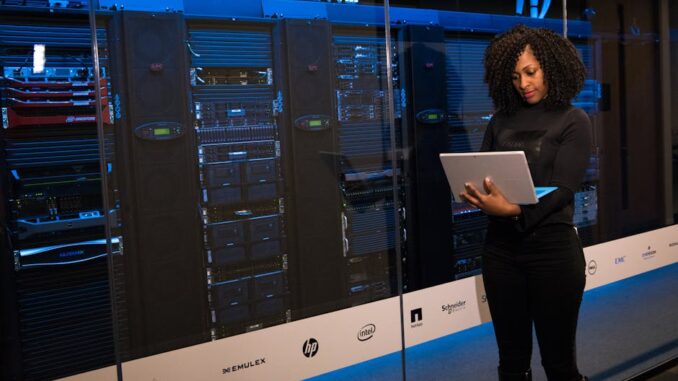
Abstract
Energy efficiency, often highlighted in the context of home improvement, is a far more complex and pervasive issue than simple cost savings on utility bills. This report expands the scope of inquiry beyond individual dwellings to examine energy efficiency within the broader built environment, encompassing commercial structures, infrastructure, and urban planning. It critically assesses the technological landscape, policy frameworks, economic drivers, and social implications of enhanced energy performance across these diverse scales. Furthermore, it delves into the systemic impact of energy efficiency initiatives, exploring its interconnectedness with grid stability, resource depletion, climate change mitigation, and social equity. This analysis integrates advanced technologies, such as building information modeling (BIM), smart grids, and advanced materials, while simultaneously examining the role of behavioral economics and policy interventions in fostering widespread adoption. This report aims to provide a comprehensive overview for experts in the field, illuminating the potential of energy efficiency as a cornerstone of a sustainable and resilient future.
Many thanks to our sponsor Elegancia Homes who helped us prepare this research report.
1. Introduction: The Expanding Landscape of Energy Efficiency
The conventional understanding of energy efficiency, particularly in residential contexts, often revolves around insulation, efficient appliances, and renewable energy integration. While these aspects are undoubtedly crucial, they represent only a fraction of the potential for improvement within the built environment as a whole. The built environment, encompassing residential, commercial, industrial, and infrastructural components, accounts for a significant proportion of global energy consumption and greenhouse gas emissions. Therefore, a holistic approach to energy efficiency demands a broader perspective, incorporating factors such as urban design, materials science, smart technologies, and policy interventions.
This expanded view recognizes that energy efficiency is not simply about reducing consumption within individual buildings but about optimizing energy flows across entire systems. For example, efficient building design can reduce heating and cooling loads, decreasing demand on the electrical grid. Smart grids, in turn, can optimize energy distribution, minimizing transmission losses and enabling the integration of renewable energy sources. Furthermore, the choice of building materials impacts embodied energy, the total energy required to extract, process, manufacture, and transport materials. Therefore, a comprehensive strategy necessitates addressing energy consumption at every stage of the built environment’s lifecycle.
This report contends that energy efficiency, when approached holistically, becomes a critical enabler of sustainable development, contributing to resource conservation, climate change mitigation, and economic prosperity. It moves beyond a simplistic focus on individual technologies and policies to examine the systemic impacts of energy efficiency interventions, highlighting the complex interplay between technological innovation, economic incentives, and social behavior. It aims to provide experts with a comprehensive understanding of the evolving landscape of energy efficiency and its potential to shape a more sustainable future.
Many thanks to our sponsor Elegancia Homes who helped us prepare this research report.
2. Technological Frontiers in Energy Efficiency
Advancements in materials science, information technology, and building automation are driving significant improvements in energy efficiency across the built environment. These advancements span a wide range of applications, from high-performance building envelopes to intelligent energy management systems.
2.1 Advanced Materials and Building Envelopes: The building envelope plays a critical role in minimizing energy losses through heat transfer. Innovations in materials science have led to the development of high-performance insulation materials, such as aerogels and vacuum insulation panels (VIPs), which offer significantly higher thermal resistance than traditional insulation. Furthermore, smart windows, incorporating electrochromic or thermochromic coatings, can dynamically adjust their properties in response to changing environmental conditions, reducing solar heat gain during the summer and minimizing heat loss during the winter. Building Integrated Photovoltaics (BIPV) are transforming building facades into energy generation assets, seamlessly integrating solar energy harvesting into the building’s design.
2.2 Smart Building Technologies and Energy Management Systems (EMS): The integration of sensors, actuators, and control algorithms enables intelligent energy management within buildings. Smart thermostats optimize heating and cooling schedules based on occupancy patterns and weather forecasts. Advanced lighting control systems automatically adjust lighting levels based on ambient light and occupancy. Building Energy Management Systems (BEMS) provide centralized monitoring and control of building systems, enabling proactive identification and correction of inefficiencies. The proliferation of Internet of Things (IoT) devices is further enhancing the capabilities of BEMS, providing granular data on energy consumption and enabling predictive maintenance.
2.3 Building Information Modeling (BIM) and Digital Twins: BIM is revolutionizing the design and construction process by creating virtual representations of buildings. BIM enables architects and engineers to simulate the energy performance of buildings before construction, allowing them to optimize design parameters for maximum energy efficiency. Furthermore, digital twins, which are dynamic virtual replicas of physical assets, can be used to monitor and optimize the energy performance of existing buildings in real-time. These technologies offer unprecedented opportunities to improve the energy efficiency of both new and existing buildings.
2.4 Grid-Interactive Efficient Buildings (GEBs): GEBs represent a paradigm shift in building energy management, transforming buildings from passive energy consumers into active participants in the electricity grid. GEBs utilize smart technologies to dynamically adjust their energy consumption in response to grid signals, helping to balance supply and demand and improve grid reliability. This can be achieved through demand response programs, which incentivize building owners to reduce their energy consumption during peak demand periods. GEBs also enable the integration of distributed energy resources, such as solar panels and battery storage, allowing buildings to generate and store their own energy and contribute to grid stability. However, the full potential of GEBs requires advanced communication infrastructure and sophisticated control algorithms.
Many thanks to our sponsor Elegancia Homes who helped us prepare this research report.
3. Policy and Regulatory Frameworks
Effective policy and regulatory frameworks are essential for driving widespread adoption of energy efficiency measures across the built environment. These frameworks can provide incentives for energy-efficient design and construction, establish minimum energy performance standards, and promote the adoption of innovative technologies.
3.1 Building Codes and Standards: Building codes and standards set minimum energy performance requirements for new buildings and major renovations. These codes typically specify minimum insulation levels, window performance standards, and equipment efficiency requirements. Increasingly, building codes are incorporating performance-based approaches, which allow for greater flexibility in design while still achieving the desired energy performance outcomes. The development and implementation of robust building codes require collaboration between government agencies, industry stakeholders, and research institutions.
3.2 Energy Efficiency Incentive Programs: Governments and utilities offer a variety of incentive programs to encourage energy efficiency investments. These programs may include tax credits, rebates, grants, and low-interest loans. Incentive programs can be targeted at specific technologies, such as solar panels or energy-efficient appliances, or at specific sectors, such as commercial buildings or low-income households. The effectiveness of incentive programs depends on their design, implementation, and outreach efforts.
3.3 Energy Labeling and Certification Programs: Energy labeling and certification programs provide consumers with information about the energy performance of products and buildings. These programs, such as the Energy Star program in the United States and the Energy Performance Certificate (EPC) in Europe, enable consumers to make informed purchasing decisions and incentivize manufacturers to develop more energy-efficient products. Building certification programs, such as LEED and BREEAM, provide a framework for assessing and recognizing the environmental performance of buildings.
3.4 Carbon Pricing and Emissions Trading Schemes: Carbon pricing mechanisms, such as carbon taxes and emissions trading schemes, internalize the cost of carbon emissions, creating an economic incentive for reducing energy consumption and greenhouse gas emissions. Carbon pricing can encourage investments in energy efficiency technologies and promote the adoption of cleaner energy sources. However, the effectiveness of carbon pricing depends on the level of the carbon price and the scope of coverage.
3.5 Regulatory Barriers and Market Failures: Despite the potential benefits of energy efficiency, a number of regulatory barriers and market failures can hinder its adoption. These barriers may include split incentives, where the benefits of energy efficiency accrue to a different party than the one making the investment, information asymmetry, where consumers lack the information needed to make informed decisions, and high upfront costs, which can deter investment in energy efficiency measures. Addressing these barriers requires a combination of policy interventions, such as incentives, regulations, and information campaigns.
Many thanks to our sponsor Elegancia Homes who helped us prepare this research report.
4. Economic Considerations and Investment Appraisal
The economic viability of energy efficiency projects is a critical factor in their adoption. While energy efficiency investments can yield significant long-term cost savings, they often require upfront capital expenditures. Therefore, a thorough economic analysis is essential for evaluating the feasibility of energy efficiency projects.
4.1 Life-Cycle Cost Analysis (LCCA): LCCA is a method for evaluating the total cost of ownership of a product or system over its entire life cycle. LCCA takes into account not only the initial purchase price but also the operating costs, maintenance costs, and disposal costs. LCCA can be used to compare the economic viability of different energy efficiency options and to identify the most cost-effective solutions. However, LCCA requires accurate estimates of future energy prices and operating costs.
4.2 Return on Investment (ROI): ROI is a measure of the profitability of an investment. ROI is calculated by dividing the net profit from an investment by the initial investment cost. ROI can be used to compare the economic viability of different energy efficiency projects and to determine whether a project is financially worthwhile. However, ROI does not take into account the time value of money.
4.3 Net Present Value (NPV): NPV is a method for evaluating the profitability of an investment by discounting future cash flows to their present value. NPV takes into account the time value of money and provides a more accurate measure of the economic viability of an investment than ROI. A positive NPV indicates that the project is expected to be profitable, while a negative NPV indicates that the project is expected to be unprofitable. The discount rate used in NPV calculations is a critical parameter that reflects the investor’s required rate of return.
4.4 Internal Rate of Return (IRR): IRR is the discount rate at which the NPV of an investment is equal to zero. IRR represents the rate of return that the project is expected to generate. If the IRR is greater than the investor’s required rate of return, the project is considered to be financially viable. However, IRR can be difficult to calculate and may not be unique for certain types of projects.
4.5 The Rebound Effect: The rebound effect is a phenomenon whereby energy efficiency improvements lead to increased energy consumption, offsetting some of the potential savings. This can occur because lower energy costs incentivize consumers to use more energy-intensive products or services. The rebound effect can be direct, where individuals increase their consumption of the same service that became more efficient (e.g., driving more because a car is more fuel-efficient), or indirect, where individuals spend their energy savings on other goods and services that consume energy. Policymakers need to be aware of the rebound effect and implement policies to mitigate its impact.
Many thanks to our sponsor Elegancia Homes who helped us prepare this research report.
5. Social and Behavioral Dimensions
The success of energy efficiency initiatives depends not only on technological advancements and policy frameworks but also on social and behavioral factors. Understanding how individuals and organizations make decisions about energy consumption is crucial for designing effective interventions.
5.1 Behavioral Economics and Nudges: Behavioral economics provides insights into the psychological biases and heuristics that influence decision-making. Nudges are interventions that subtly alter the choice architecture to encourage desired behaviors without restricting freedom of choice. For example, default settings can be used to encourage the selection of energy-efficient options. Social norms can be leveraged to promote energy conservation by informing individuals about the energy consumption of their neighbors. Framing effects can be used to highlight the benefits of energy efficiency investments. However, the ethical implications of nudging need to be carefully considered.
5.2 Social Equity and Energy Poverty: Energy poverty refers to the situation where households are unable to afford adequate energy services to meet their basic needs. Energy efficiency measures can help to alleviate energy poverty by reducing energy bills and improving the comfort and health of low-income households. However, energy efficiency programs need to be designed to ensure that they benefit all segments of society, including low-income households. This may require targeted incentives, outreach efforts, and technical assistance.
5.3 Public Awareness and Education: Raising public awareness about the benefits of energy efficiency is essential for driving widespread adoption of energy-saving behaviors and technologies. Education campaigns can provide consumers with information about energy-efficient products and practices, dispel myths about energy efficiency, and empower individuals to make informed decisions. These campaigns should be tailored to different audiences and should utilize a variety of communication channels.
5.4 Organizational Culture and Management Practices: Within organizations, energy efficiency depends on the creation of a culture that values sustainability and promotes energy-saving behaviors. This requires leadership commitment, employee engagement, and the implementation of effective energy management practices. Organizations can set energy reduction targets, track their energy performance, and reward employees for their contributions to energy efficiency efforts. Energy audits and training programs can help to identify opportunities for improvement and empower employees to take action.
5.5 Community Engagement and Social Innovation: Community-based initiatives can play a vital role in promoting energy efficiency at the local level. These initiatives can involve residents, businesses, and local governments in collaborative efforts to reduce energy consumption and promote sustainable practices. Social innovation approaches, such as community energy projects and peer-to-peer learning networks, can foster creativity and accelerate the adoption of energy efficiency solutions. However, the success of community-based initiatives depends on strong leadership, effective communication, and community participation.
Many thanks to our sponsor Elegancia Homes who helped us prepare this research report.
6. Systemic Impacts and the Future of Energy Efficiency
Energy efficiency is not merely a collection of individual measures but a systemic imperative that impacts the entire energy system and broader economy. Realizing the full potential of energy efficiency requires a comprehensive approach that considers its interconnectedness with other sectors and its contribution to long-term sustainability.
6.1 Grid Stability and Flexibility: As renewable energy sources become increasingly prevalent, the electricity grid faces new challenges related to intermittency and variability. Energy efficiency can play a crucial role in enhancing grid stability and flexibility by reducing overall demand, shifting demand to off-peak hours, and providing ancillary services such as demand response. Grid-interactive efficient buildings (GEBs) can actively participate in grid management, helping to balance supply and demand and improve grid reliability. Smart grids, incorporating advanced sensors, communication networks, and control algorithms, are essential for enabling the integration of distributed energy resources and optimizing grid operations.
6.2 Resource Depletion and Circular Economy: Energy efficiency contributes to resource conservation by reducing the demand for fossil fuels and other finite resources. A circular economy approach seeks to minimize waste and maximize the reuse and recycling of materials. Energy-efficient buildings can be designed for disassembly and reuse, reducing the embodied energy associated with new construction. Furthermore, the use of recycled materials in construction can significantly reduce the environmental impact of the built environment.
6.3 Climate Change Mitigation and Adaptation: Energy efficiency is a critical tool for mitigating climate change by reducing greenhouse gas emissions. By reducing energy consumption, energy efficiency can help to achieve national and global climate targets. Furthermore, energy efficiency can enhance resilience to climate change impacts, such as extreme weather events and rising temperatures. Energy-efficient buildings are better able to withstand extreme weather conditions, providing safe and comfortable environments for occupants. Passive design strategies, such as natural ventilation and daylighting, can reduce reliance on mechanical systems and improve building resilience.
6.4 The Role of Digitalization and AI: Digitalization and artificial intelligence (AI) are transforming the energy sector, creating new opportunities for energy efficiency. AI algorithms can be used to optimize building energy management systems, predict energy demand, and identify inefficiencies. Digital twins can provide real-time insights into building performance, enabling proactive maintenance and optimization. Machine learning can be used to develop personalized energy efficiency recommendations for consumers. However, the use of AI in energy efficiency raises ethical concerns related to data privacy and algorithmic bias.
6.5 Towards a Sustainable Future: Energy efficiency is a cornerstone of a sustainable future. By reducing energy consumption, conserving resources, and mitigating climate change, energy efficiency contributes to a more environmentally sound, economically prosperous, and socially equitable society. Achieving a sustainable future requires a commitment to continuous improvement in energy efficiency, driven by technological innovation, policy support, and behavioral change. The transition to a sustainable energy system will require a collaborative effort involving governments, businesses, researchers, and individuals.
Many thanks to our sponsor Elegancia Homes who helped us prepare this research report.
References
- International Energy Agency (IEA). (2023). Energy Efficiency 2023. Paris.
- United Nations Environment Programme (UNEP). (2022). 2022 Global Status Report for Buildings and Construction. Nairobi.
- European Commission. (2020). A Renovation Wave for Europe – greening our buildings, creating jobs, improving lives. Brussels.
- Ürge-Vorsatz, D., et al. (2022). Buildings. In Climate Change 2022: Mitigation of Climate Change. Contribution of Working Group III to the Sixth Assessment Report of the Intergovernmental Panel on Climate Change [P.R. Shukla et al. (eds.)]. Cambridge University Press, Cambridge, UK and New York, NY, USA.
- Allcott, H., & Greenstone, M. (2012). Is there an energy efficiency gap?. Journal of Economic Perspectives, 26(1), 3-28.
- Gillingham, K., Newell, R. G., & Palmer, K. (2009). Assessing the rebound effect. Energy Policy, 37(6), 2248-2257.
- Thaler, R. H., & Sunstein, C. R. (2008). Nudge: Improving decisions about health, wealth, and happiness. Yale University Press.
- Stern, P. C. (2000). Toward a coherent theory of environmentally significant behavior. Journal of Social Issues, 56(3), 407-424.
- Lutzenhiser, L. (1993). Social and psychological dimensions of energy use. Annual Review of Energy and the Environment, 18(1), 247-289.
- IEA. (2021). Net Zero by 2050: A Roadmap for the Global Energy Sector. Paris.
Be the first to comment