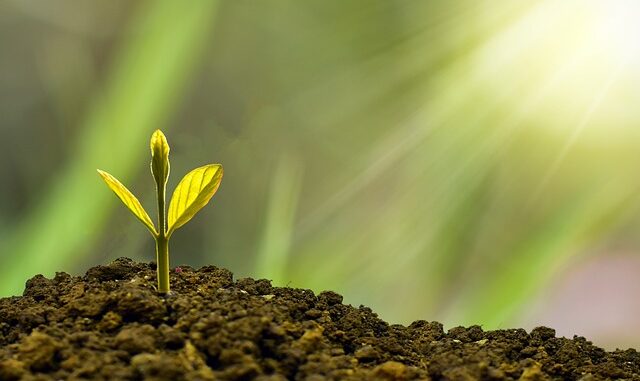
Horticultural Innovation: Advancing Plant Science and Sustainable Practices in the 21st Century
Abstract
Horticulture, encompassing the art and science of cultivating plants, is undergoing a significant transformation driven by technological advancements, climate change challenges, and evolving consumer demands. This research report explores key areas of innovation within horticulture, focusing on plant breeding and genetic engineering, controlled environment agriculture (CEA), sustainable practices, and the integration of data analytics and precision technologies. It examines the potential benefits and challenges associated with each area, emphasizing the need for interdisciplinary collaboration and a holistic approach to ensure the long-term sustainability and resilience of horticultural systems. The report highlights the importance of adapting to changing environmental conditions, promoting biodiversity, and optimizing resource utilization to meet the growing global demand for high-quality, nutritious, and sustainably produced horticultural crops.
1. Introduction: The Evolving Landscape of Horticulture
Horticulture, derived from the Latin words hortus (garden) and cultura (cultivation), has historically been viewed as a practice rooted in tradition and empirical knowledge. However, the 21st century has witnessed a rapid evolution in the field, driven by scientific advancements, technological innovation, and pressing global challenges such as climate change, food security, and resource scarcity. While traditional horticultural practices remain valuable, the integration of cutting-edge research and technology is crucial for enhancing crop productivity, improving quality, and promoting environmental sustainability.
The scope of horticulture is broad, encompassing the cultivation of fruits, vegetables, ornamental plants, herbs, and other crops. It plays a vital role in providing food, improving human health and well-being, enhancing the aesthetic appeal of landscapes, and contributing to economic development. The global horticulture market is substantial and continues to grow, driven by increasing consumer demand for fresh produce, organic products, and locally sourced food.
This report examines several key areas of innovation within horticulture, including plant breeding and genetic engineering, controlled environment agriculture, sustainable practices, and the integration of data analytics and precision technologies. These areas represent significant opportunities for advancing plant science, optimizing resource utilization, and addressing the challenges of a changing world. The aim is to provide an overview of current research trends and future directions, highlighting the importance of interdisciplinary collaboration and a holistic approach to ensure the long-term sustainability and resilience of horticultural systems.
2. Plant Breeding and Genetic Engineering: Enhancing Crop Performance
Plant breeding has been a cornerstone of horticultural development for centuries, allowing growers to select and propagate plants with desirable traits such as high yield, disease resistance, and improved fruit quality. Traditional breeding methods involve crossing different plant varieties and selecting offspring with the desired characteristics. However, these methods can be time-consuming and limited by the genetic compatibility of different species.
Genetic engineering, also known as genetic modification (GM), offers a more precise and efficient approach to plant improvement. It involves directly introducing specific genes into a plant’s genome to confer desirable traits. GM technology has been used to develop crops with enhanced pest resistance (e.g., Bt corn and cotton), herbicide tolerance (e.g., Roundup Ready soybeans), and improved nutritional content (e.g., Golden Rice). The use of CRISPR-Cas9 gene editing is a more recent development that allows for even more targeted and precise modifications of plant genomes, and avoids introducing foreign genes, thereby reducing regulatory scrutiny in some jurisdictions.
The application of genetic engineering in horticulture has the potential to revolutionize crop production. For example, researchers are developing GM fruits and vegetables with enhanced flavor, extended shelf life, and increased tolerance to environmental stresses such as drought and salinity. Furthermore, GM technology can be used to improve the nutritional value of horticultural crops, addressing micronutrient deficiencies in developing countries.
Despite the potential benefits, genetic engineering remains a controversial topic. Concerns have been raised about the potential environmental impacts of GM crops, such as the development of herbicide-resistant weeds and the unintended effects on non-target organisms. Consumer acceptance of GM foods is also a major challenge, with some consumers expressing concerns about safety and labeling. Stringent regulatory frameworks are in place to assess the safety of GM crops before they are released for commercial cultivation. Transparency and public engagement are essential for building trust and addressing public concerns about GM technology. The scientific community is also divided with some scientists pointing to the lack of evidence of harm from GMOs in general, and others highlighting the need for long term independent safety assessments.
3. Controlled Environment Agriculture (CEA): Optimizing Growing Conditions
Controlled environment agriculture (CEA) encompasses a range of technologies and practices that allow growers to precisely control environmental factors such as temperature, humidity, light, carbon dioxide concentration, and nutrient supply. CEA systems include greenhouses, vertical farms, and plant factories. These systems offer several advantages over traditional open-field agriculture, including increased yields, reduced water consumption, improved pest and disease control, and the ability to grow crops year-round, regardless of climate conditions.
Greenhouses are the most widely used form of CEA. They provide a protective environment for crops, allowing growers to extend the growing season and produce high-quality crops in a controlled environment. Modern greenhouses are equipped with sophisticated climate control systems that can automatically adjust temperature, humidity, and ventilation based on real-time sensor data.
Vertical farms represent a more advanced form of CEA. These systems involve growing crops in vertically stacked layers, often indoors, using artificial lighting and hydroponic or aeroponic nutrient delivery systems. Vertical farms can achieve extremely high yields per unit area and can be located in urban areas, reducing transportation costs and improving access to fresh produce. However, vertical farms require significant capital investment and energy inputs, particularly for lighting.
Plant factories are the most technologically advanced form of CEA. These systems are completely enclosed and automated, with precise control over all environmental factors. Plant factories can achieve the highest levels of productivity and resource efficiency, but they also require the highest capital investment and energy inputs. LEDs are becoming more commonplace than traditional lighting systems due to their lower energy requirements and the ability to fine-tune the light spectrum.
CEA technologies offer significant potential for enhancing horticultural production in the face of climate change and resource scarcity. However, optimizing the energy efficiency of CEA systems is crucial for their long-term sustainability. Research is focused on developing more efficient lighting systems, improving insulation, and utilizing renewable energy sources to power CEA facilities.
4. Sustainable Practices: Minimizing Environmental Impact
Horticulture has the potential to contribute to environmental sustainability through the adoption of practices that minimize the use of resources, reduce pollution, and enhance biodiversity. Sustainable horticultural practices include integrated pest management (IPM), water conservation, soil health management, and organic farming.
Integrated pest management (IPM) is an approach to pest control that emphasizes prevention and monitoring, using pesticides only as a last resort. IPM involves a combination of cultural practices, biological control, and targeted pesticide applications to minimize the impact on beneficial insects and the environment. Biological control involves using natural enemies of pests, such as predatory insects and parasitic wasps, to control pest populations. Cultural practices such as crop rotation, sanitation, and proper irrigation can also help to prevent pest outbreaks.
Water conservation is essential for sustainable horticulture, particularly in arid and semi-arid regions. Drip irrigation and micro-sprinklers deliver water directly to the roots of plants, minimizing water loss through evaporation and runoff. Rainwater harvesting and greywater recycling can also be used to supplement irrigation water supplies. The development of drought-tolerant crop varieties is another important strategy for reducing water consumption in horticulture.
Soil health management focuses on improving the physical, chemical, and biological properties of soil. Practices such as cover cropping, no-till farming, and the application of compost and organic amendments can enhance soil fertility, improve water infiltration, and reduce erosion. Healthy soils also support a diverse community of soil organisms, which play a vital role in nutrient cycling and disease suppression.
Organic farming is a holistic approach to crop production that prohibits the use of synthetic pesticides, fertilizers, and genetically modified organisms. Organic farming relies on natural processes such as crop rotation, composting, and biological pest control to maintain soil fertility and control pests and diseases. Organic farming practices can enhance biodiversity and reduce the environmental impact of agriculture, but yields may be lower compared to conventional farming systems. While yields may be lower, the produce can often command a higher price in retail markets and is a rapidly growing trend in the food sector.
5. Data Analytics and Precision Technologies: Optimizing Resource Utilization
The integration of data analytics and precision technologies is transforming horticultural practices, enabling growers to optimize resource utilization, improve crop quality, and reduce environmental impact. Precision agriculture involves using sensors, GPS, and other technologies to collect real-time data on soil conditions, plant health, and environmental factors. This data can be analyzed to make informed decisions about irrigation, fertilization, and pest control.
Remote sensing technologies, such as drones and satellites, can be used to monitor crop health and identify areas of stress or disease. Spectral imaging can detect subtle changes in plant reflectance that are indicative of nutrient deficiencies or pest infestations. This information can be used to target interventions and optimize resource allocation.
Artificial intelligence (AI) and machine learning are being used to develop predictive models that can forecast crop yields, optimize irrigation schedules, and detect early signs of disease. AI can also be used to automate tasks such as weed detection and harvesting, reducing labor costs and improving efficiency. The increasing use of robotics for harvesting is a particularly exciting field, as it addresses the challenge of finding skilled labor for physically demanding tasks.
Data analytics and precision technologies can help growers to optimize resource utilization, reduce environmental impact, and improve crop quality. However, the adoption of these technologies requires significant investment in infrastructure and training. Furthermore, data privacy and security are important considerations, as growers may be reluctant to share sensitive data with third-party providers.
6. Addressing Challenges and Future Directions
While the innovations discussed in this report offer significant potential for advancing horticulture, several challenges must be addressed to ensure their successful implementation and long-term sustainability. These challenges include:
- Climate Change: Climate change poses a significant threat to horticultural production, with increasing temperatures, changing precipitation patterns, and more frequent extreme weather events. Developing climate-resilient crop varieties and adapting horticultural practices to mitigate the impacts of climate change are crucial for ensuring food security.
- Resource Scarcity: Water, land, and energy are becoming increasingly scarce resources. Optimizing resource utilization and minimizing environmental impact are essential for sustainable horticulture. This includes adopting water-efficient irrigation techniques, improving soil health, and utilizing renewable energy sources.
- Pest and Disease Management: Pests and diseases can cause significant losses in horticultural crops. Developing integrated pest management strategies that minimize the use of pesticides and promote biological control is crucial for protecting crop yields and reducing environmental impact.
- Consumer Acceptance: Consumer acceptance of new technologies, such as genetic engineering and vertical farming, is essential for their widespread adoption. Transparency, public engagement, and effective communication are crucial for building trust and addressing consumer concerns.
- Regulatory Frameworks: Regulatory frameworks must be flexible and responsive to new technologies while ensuring that they are safe for human health and the environment. Collaboration between researchers, regulators, and industry stakeholders is essential for developing effective regulatory policies.
Looking ahead, several key areas of research and development will be crucial for advancing horticulture in the 21st century. These include:
- Developing Climate-Resilient Crops: Breeding and genetic engineering can be used to develop crops that are more tolerant to drought, heat, salinity, and other environmental stresses.
- Improving Resource Efficiency: Research is needed to develop more efficient irrigation systems, optimize nutrient management, and reduce energy consumption in CEA facilities.
- Enhancing Soil Health: Improving soil health through practices such as cover cropping, composting, and reduced tillage can enhance soil fertility, improve water infiltration, and reduce erosion.
- Developing Sustainable Pest Management Strategies: Integrated pest management strategies that minimize the use of pesticides and promote biological control are essential for protecting crop yields and reducing environmental impact.
- Optimizing CEA Systems: Research is needed to optimize the design and operation of CEA systems, including greenhouses, vertical farms, and plant factories, to improve energy efficiency and reduce costs.
- Integrating Data Analytics and Precision Technologies: The integration of data analytics and precision technologies can help growers to optimize resource utilization, improve crop quality, and reduce environmental impact.
7. Conclusion
Horticulture is undergoing a period of rapid innovation, driven by scientific advancements, technological innovation, and pressing global challenges. Plant breeding and genetic engineering, controlled environment agriculture, sustainable practices, and the integration of data analytics and precision technologies offer significant potential for enhancing crop productivity, improving quality, and promoting environmental sustainability. The successful implementation of these innovations requires interdisciplinary collaboration, a holistic approach, and a commitment to addressing the challenges of climate change, resource scarcity, and consumer acceptance. By embracing innovation and adopting sustainable practices, horticulture can play a vital role in ensuring food security, improving human health and well-being, and protecting the environment for future generations.
References
- Albrecht, A., & Ramchurn, S. D. (2022). Artificial intelligence for sustainable agriculture. Philosophical Transactions of the Royal Society B, 377(1847), 20210185.
- FAO. (2017). The future of food and agriculture – Trends and challenges. Food and Agriculture Organization of the United Nations.
- Godfray, H. C. J., Beddington, J. R., Crute, I. R., Haddad, L., Lawrence, D., Muir, J. F., … & Toulmin, C. (2010). Food security: the challenge of feeding 9 billion people. Science, 327(5967), 812-818.
- Jones, N. (2015). The first gene-edited crops are here. Nature, 522(7556), 413-414.
- Kozai, T., Fujiwara, K., Runkle, E. S., & Both, A. J. (Eds.). (2020). LED Lighting for Urban Agriculture. Springer.
- Pérez-Jiménez, J., Díaz-Rubio, M. E., Ibáñez-Hernández, M. D., & Saura-Calixto, F. (2021). The influence of organic production on fruit and vegetable nutritional quality: A systematic review and meta-analysis. Critical Reviews in Food Science and Nutrition, 61(17), 2870-2891.
- Savvas, D., & Gruda, N. (2018). Nutrient uptake and plant nutrition in modern soilless horticulture. In Soilless Culture (pp. 49-113). Academic Press.
- van Delden, S. H., Khondoker, M. R. H., de Gelder, A., Marcelis, L. F. M., & Stanghellini, C. (2021). A simulation model for evaluating sustainable energy systems for vertical farms. Renewable and Sustainable Energy Reviews, 137, 110474.
- Whitaker, M., Hilton, H. W., Ferrero, A., Duke, S. O., Dayan, F. E., & Holst, D. C. (2024). Herbicide resistance: cause, effect, and mitigation. Pest Management Science, 80(1), 14-32.
Be the first to comment