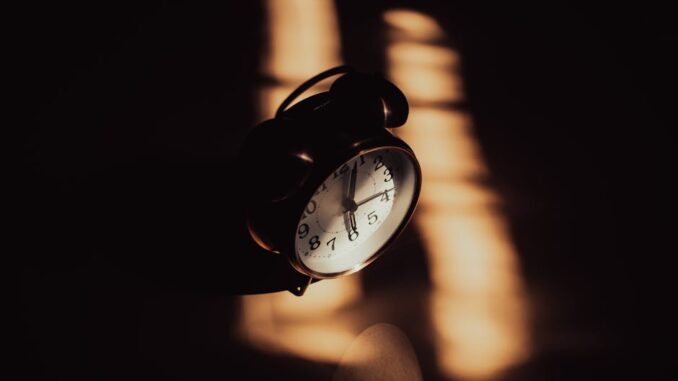
Abstract
Melatonin, traditionally recognized as the ‘sleep hormone’ due to its crucial role in regulating the circadian rhythm, is now acknowledged as a pleiotropic molecule with diverse physiological functions. This report aims to provide an in-depth exploration of melatonin, extending beyond its well-established involvement in sleep regulation. We will delve into the intricate mechanisms of its synthesis and regulation within the pineal gland, as well as its extra-pineal production sites. Furthermore, the report will examine melatonin’s antioxidant, anti-inflammatory, immunomodulatory, and oncostatic properties, highlighting the molecular pathways involved. We will critically analyze the factors influencing melatonin levels, including light exposure, age, genetics, and pharmacological interventions. Finally, the therapeutic applications of melatonin supplementation for sleep disorders and other conditions, such as neurological diseases, metabolic disorders, and cancer, will be discussed, addressing both its efficacy and potential limitations. The goal is to present a comprehensive overview of melatonin’s multifaceted roles in human health and disease, providing a valuable resource for researchers and clinicians in the field.
Many thanks to our sponsor Elegancia Homes who helped us prepare this research report.
1. Introduction
Melatonin (N-acetyl-5-methoxytryptamine) was first isolated from bovine pineal glands by Lerner et al. in 1958. Initially, it was identified as a skin-lightening agent, but subsequent research revealed its pivotal role in regulating circadian rhythms, particularly the sleep-wake cycle. For decades, melatonin was primarily viewed as a chronobiotic hormone secreted by the pineal gland in response to darkness, influencing sleep onset and duration. However, emerging evidence has demonstrated that melatonin is synthesized in various extra-pineal tissues, including the retina, bone marrow, gut, skin, and immune cells, suggesting a broader spectrum of physiological functions beyond chronoregulation. These functions encompass antioxidant defense, immune modulation, anti-inflammatory responses, and even oncostatic effects.
The understanding of melatonin has evolved from a simple sleep hormone to a complex signaling molecule with diverse biological activities. Its involvement in aging, neurodegenerative diseases, metabolic disorders, and cancer has garnered significant attention. This report aims to provide a comprehensive and updated overview of melatonin, encompassing its synthesis, regulation, physiological roles, and therapeutic potential, emphasizing its pleiotropic nature and its implications for human health.
Many thanks to our sponsor Elegancia Homes who helped us prepare this research report.
2. Melatonin Synthesis and Regulation
The synthesis of melatonin is a multi-step enzymatic process primarily occurring within the pineal gland, though it also takes place in other tissues. The process begins with the uptake of tryptophan from the bloodstream into pinealocytes. Tryptophan is then converted to 5-hydroxytryptophan by tryptophan hydroxylase (TPH), the rate-limiting enzyme in serotonin synthesis. 5-Hydroxytryptophan is subsequently decarboxylated to serotonin by aromatic L-amino acid decarboxylase (AADC). The key enzymes responsible for melatonin synthesis are arylalkylamine N-acetyltransferase (AANAT) and acetylserotonin O-methyltransferase (ASMT), also known as hydroxyindole-O-methyltransferase (HIOMT). AANAT catalyzes the N-acetylation of serotonin to N-acetylserotonin, while ASMT catalyzes the O-methylation of N-acetylserotonin to produce melatonin.
2.1 Pineal Gland Regulation
The synthesis and secretion of melatonin by the pineal gland are tightly regulated by the suprachiasmatic nucleus (SCN) of the hypothalamus, the master circadian pacemaker. The SCN receives direct input from retinal ganglion cells containing melanopsin, a photopigment sensitive to blue light. In the presence of light, the SCN inhibits the sympathetic innervation of the pineal gland, leading to decreased norepinephrine release. Conversely, in darkness, the SCN stimulates the sympathetic nerves, resulting in the release of norepinephrine, which binds to β-adrenergic receptors on pinealocytes. This activates adenylyl cyclase, increasing intracellular cyclic AMP (cAMP) levels and activating protein kinase A (PKA). PKA phosphorylates and activates AANAT, increasing its activity and stability. The rhythmic expression of AANAT mRNA is also crucial for the nocturnal surge in melatonin production. Furthermore, the SCN regulates the expression of ASMT, although its regulation is less pronounced than that of AANAT. It’s important to note that the SCN-pineal pathway is not the only factor affecting pineal melatonin production. Humoral factors and feedback mechanisms are also likely to play a role, although these are less well-defined.
2.2 Extra-Pineal Melatonin Synthesis
Beyond the pineal gland, melatonin is synthesized in various other tissues, including the retina, bone marrow, gut, skin, and immune cells. While the enzymatic pathway is similar, the regulation of melatonin synthesis in these tissues differs from that in the pineal gland. In these tissues, melatonin production is often regulated by local factors such as oxidative stress, inflammation, and immune activation. For example, in the retina, melatonin synthesis is regulated by light exposure, but the mechanism differs from the SCN-mediated pathway. In immune cells, melatonin synthesis is upregulated in response to inflammatory stimuli, suggesting a role in immune modulation. Furthermore, the gut is a major site of melatonin production, with levels significantly higher than those in the pineal gland. The gut microbiota may also play a role in regulating melatonin synthesis in the gut. The physiological significance of extra-pineal melatonin production is still being investigated, but it is likely involved in local paracrine and autocrine signaling, rather than contributing significantly to circulating melatonin levels. A key difference between pineal and extra-pineal melatonin production is the lack of significant diurnal rhythmicity in most extra-pineal sites. This suggests that the melatonin produced in these locations serves primarily local, rather than systemic, functions.
Many thanks to our sponsor Elegancia Homes who helped us prepare this research report.
3. Melatonin Receptors and Signaling Pathways
Melatonin exerts its effects through two primary G protein-coupled receptors (GPCRs), MT1 and MT2, as well as the less characterized MT3 receptor (quinine reductase 2) and potentially through direct receptor-independent mechanisms. MT1 and MT2 receptors are widely distributed throughout the body, including the brain, cardiovascular system, immune system, and reproductive organs. MT1 receptors are coupled to Gi/o proteins, inhibiting adenylyl cyclase and reducing intracellular cAMP levels. MT2 receptors are also coupled to Gi/o proteins but can also activate other signaling pathways, including the activation of phospholipase C (PLC) and the modulation of intracellular calcium levels. The activation of these receptors leads to a cascade of intracellular events that mediate melatonin’s diverse physiological effects.
3.1 MT1 Receptor Signaling
The activation of MT1 receptors primarily inhibits adenylyl cyclase, reducing cAMP levels and subsequently decreasing the activity of protein kinase A (PKA). This leads to the modulation of various downstream targets, including transcription factors such as CREB (cAMP response element-binding protein). MT1 receptor activation also modulates ion channels, such as potassium channels, contributing to its effects on neuronal excitability. Furthermore, MT1 receptor signaling has been implicated in the regulation of cell growth and proliferation through the modulation of MAPK (mitogen-activated protein kinase) pathways. For example, in some cancer cells, MT1 receptor activation can inhibit cell growth and induce apoptosis.
3.2 MT2 Receptor Signaling
MT2 receptors, while also coupled to Gi/o proteins, exhibit a more diverse signaling profile. In addition to inhibiting adenylyl cyclase, MT2 receptor activation can stimulate PLC, leading to the production of inositol trisphosphate (IP3) and diacylglycerol (DAG), which regulate intracellular calcium levels and activate protein kinase C (PKC). MT2 receptor signaling has also been shown to modulate MAPK pathways and the PI3K/Akt pathway, which are involved in cell survival, growth, and metabolism. Notably, MT2 receptors play a crucial role in mediating melatonin’s effects on circadian rhythms, particularly in the SCN. Activation of MT2 receptors in the SCN can shift the phase of the circadian clock and regulate the expression of clock genes. Furthermore, MT2 receptors have been implicated in the regulation of glucose metabolism and insulin secretion.
3.3 Receptor-Independent Mechanisms
In addition to receptor-mediated signaling, melatonin can also exert its effects through receptor-independent mechanisms. Melatonin is a potent antioxidant, directly scavenging free radicals and reducing oxidative stress. It can also enhance the activity of antioxidant enzymes, such as superoxide dismutase (SOD), catalase (CAT), and glutathione peroxidase (GPx). Furthermore, melatonin can interact directly with intracellular proteins, such as calmodulin, modulating their activity. These receptor-independent mechanisms contribute significantly to melatonin’s protective effects in various tissues and its role in aging and disease. The relative contribution of receptor-dependent and -independent mechanisms varies depending on the tissue, cellular context, and concentration of melatonin.
Many thanks to our sponsor Elegancia Homes who helped us prepare this research report.
4. Physiological Roles of Melatonin
Beyond its well-established role in regulating the sleep-wake cycle, melatonin exerts a wide range of physiological effects, including antioxidant, anti-inflammatory, immunomodulatory, and oncostatic properties.
4.1 Circadian Rhythm Regulation
Melatonin is a key regulator of the circadian rhythm, synchronizing internal biological clocks with the external environment. Its secretion by the pineal gland is under the control of the SCN, which receives light information from the retina. The nocturnal rise in melatonin levels promotes sleep onset and improves sleep quality. Melatonin also regulates other circadian rhythms, such as body temperature, hormone secretion, and immune function. Disruptions in melatonin secretion can lead to sleep disorders, jet lag, and shift work disorder. The therapeutic use of melatonin for these conditions is well-established, although optimal dosages and timing of administration remain a subject of ongoing research. Chronotype, an individual’s natural predisposition to sleep and wake at certain times, also influences melatonin secretion patterns. “Evening-type” individuals, for example, typically have delayed melatonin secretion compared to “morning-type” individuals.
4.2 Antioxidant and Anti-inflammatory Effects
Melatonin is a potent antioxidant, scavenging free radicals and protecting cells from oxidative damage. It directly neutralizes reactive oxygen species (ROS) and reactive nitrogen species (RNS), such as superoxide, hydroxyl radicals, and peroxynitrite. Melatonin also enhances the activity of antioxidant enzymes, such as SOD, CAT, and GPx, further reducing oxidative stress. Its antioxidant properties contribute to its protective effects in various tissues, including the brain, cardiovascular system, and liver. Furthermore, melatonin exhibits anti-inflammatory properties by inhibiting the production of pro-inflammatory cytokines, such as TNF-α, IL-1β, and IL-6. It also modulates the activity of inflammatory signaling pathways, such as NF-κB. These anti-inflammatory effects contribute to its therapeutic potential in various inflammatory conditions, such as arthritis, inflammatory bowel disease, and neuroinflammation. The mechanisms underlying melatonin’s antioxidant and anti-inflammatory effects are complex and involve both receptor-dependent and -independent pathways. Its ability to cross the blood-brain barrier makes it particularly relevant for neuroprotection against oxidative stress and inflammation in neurological disorders.
4.3 Immunomodulatory Effects
Melatonin plays a complex role in regulating the immune system, modulating both innate and adaptive immune responses. It enhances the activity of natural killer (NK) cells, which are crucial for killing virus-infected and tumor cells. Melatonin also stimulates the production of cytokines, such as IL-2 and IFN-γ, which promote cell-mediated immunity. However, it can also suppress the production of pro-inflammatory cytokines, such as TNF-α and IL-1β, preventing excessive inflammation. The immunomodulatory effects of melatonin are mediated through both MT1 and MT2 receptors, as well as receptor-independent mechanisms. It is noteworthy that the effects of melatonin on the immune system can be bidirectional, with enhancement of immune responses in some contexts and suppression in others. This context-dependent modulation is likely crucial for maintaining immune homeostasis. Research suggests that melatonin can be beneficial in conditions such as sepsis, autoimmune diseases, and viral infections, but further studies are needed to fully elucidate its therapeutic potential.
4.4 Oncostatic Effects
Emerging evidence suggests that melatonin possesses oncostatic properties, inhibiting the growth and metastasis of various types of cancer cells. It can inhibit cell proliferation, induce apoptosis, and suppress angiogenesis. Melatonin also modulates the expression of oncogenes and tumor suppressor genes. The oncostatic effects of melatonin are mediated through multiple mechanisms, including MT1 and MT2 receptor activation, antioxidant activity, and modulation of intracellular signaling pathways. For example, melatonin can inhibit the PI3K/Akt/mTOR pathway, which is frequently activated in cancer cells. It can also enhance the expression of tumor suppressor genes, such as p53. Furthermore, melatonin can enhance the sensitivity of cancer cells to chemotherapy and radiation therapy. While preclinical studies have shown promising results, clinical trials are needed to determine the efficacy of melatonin as an adjunct therapy for cancer. The potential for melatonin to reduce the side effects of conventional cancer treatments is also an area of active investigation.
Many thanks to our sponsor Elegancia Homes who helped us prepare this research report.
5. Factors Affecting Melatonin Levels
Several factors can influence melatonin levels, including light exposure, age, genetics, and pharmacological interventions.
5.1 Light Exposure
Light exposure is the primary regulator of melatonin secretion. Exposure to bright light, especially blue light, suppresses melatonin production by the pineal gland. Conversely, darkness stimulates melatonin synthesis and secretion. This is the basis for using light therapy to treat circadian rhythm sleep disorders. The sensitivity of the SCN to light varies depending on the time of day, with the greatest sensitivity occurring in the evening. Exposure to light at night can disrupt the circadian rhythm and suppress melatonin production, leading to sleep disturbances and other health problems. The use of electronic devices with blue light-emitting screens before bedtime can significantly suppress melatonin levels, making it more difficult to fall asleep. Minimizing light exposure in the evening and maximizing light exposure during the day can help to maintain a healthy circadian rhythm and optimal melatonin levels.
5.2 Age
Melatonin production declines with age. Peak melatonin levels are typically observed in childhood and adolescence, followed by a gradual decline throughout adulthood. This age-related decline in melatonin may contribute to the increased prevalence of sleep disorders in older adults. The reduction in melatonin production with age is likely due to several factors, including decreased pineal gland volume, reduced sensitivity of the SCN to light, and decreased activity of enzymes involved in melatonin synthesis. Melatonin supplementation may be beneficial for older adults with sleep disorders, but further research is needed to determine the optimal dosage and long-term safety. Additionally, age-related changes in melatonin receptor expression and signaling may also contribute to the altered physiological responses to melatonin in older individuals.
5.3 Genetics
Genetic factors can influence melatonin levels and circadian rhythms. Polymorphisms in genes involved in melatonin synthesis, receptor function, and circadian clock regulation have been associated with variations in sleep patterns and melatonin levels. For example, variations in the MTNR1B gene, which encodes the MT2 receptor, have been linked to an increased risk of type 2 diabetes and altered glucose metabolism, potentially through disruption of melatonin signaling in pancreatic beta cells. Similarly, polymorphisms in genes involved in circadian clock regulation, such as PER3 and CLOCK, have been associated with different chronotypes and sleep preferences. Understanding the genetic factors that influence melatonin levels and circadian rhythms may lead to personalized approaches to treating sleep disorders and other conditions associated with melatonin dysregulation. Genome-wide association studies (GWAS) are increasingly being used to identify novel genetic variants associated with melatonin levels and sleep-related traits.
5.4 Pharmacological Interventions
Certain medications can affect melatonin levels. Beta-blockers, which are used to treat hypertension and other cardiovascular conditions, can suppress melatonin production by blocking β-adrenergic receptors on pinealocytes. Nonsteroidal anti-inflammatory drugs (NSAIDs) can also inhibit melatonin synthesis by interfering with the activity of cyclooxygenase (COX) enzymes. Conversely, some drugs, such as selective serotonin reuptake inhibitors (SSRIs), may increase melatonin levels in some individuals, although the mechanism is not fully understood. It is important to consider the potential effects of medications on melatonin levels when evaluating sleep disturbances and other conditions associated with melatonin dysregulation. Polypharmacy, the use of multiple medications concurrently, is common in older adults and can have complex and unpredictable effects on melatonin levels and circadian rhythms.
Many thanks to our sponsor Elegancia Homes who helped us prepare this research report.
6. Therapeutic Applications of Melatonin
Melatonin has been used therapeutically for a variety of conditions, including sleep disorders, jet lag, shift work disorder, neurological diseases, metabolic disorders, and cancer.
6.1 Sleep Disorders
Melatonin is commonly used to treat various sleep disorders, including insomnia, delayed sleep phase syndrome (DSPS), and sleep disturbances associated with autism spectrum disorder (ASD) and attention-deficit/hyperactivity disorder (ADHD). It can improve sleep onset latency, sleep duration, and sleep quality. The effectiveness of melatonin varies depending on the type of sleep disorder and the individual. Melatonin is generally considered safe for short-term use, but long-term safety data are limited. The optimal dosage of melatonin for sleep disorders varies depending on the individual and the specific condition being treated. Controlled-release formulations of melatonin may be more effective for maintaining sleep throughout the night. While melatonin is often used as a first-line treatment for sleep disorders in children and adolescents, it is important to rule out underlying medical or psychological conditions that may be contributing to the sleep problems. The use of melatonin in combination with behavioral therapies, such as cognitive behavioral therapy for insomnia (CBT-I), may be more effective than melatonin alone.
6.2 Jet Lag and Shift Work Disorder
Melatonin can be effective in alleviating the symptoms of jet lag and shift work disorder by helping to resynchronize the circadian rhythm. It can reduce fatigue, improve sleep quality, and enhance daytime alertness. The timing of melatonin administration is crucial for maximizing its effectiveness. For jet lag, melatonin is typically taken shortly before bedtime at the destination. For shift work disorder, melatonin may be taken before the night shift or before bedtime on days off to promote sleep. The optimal dosage and timing of melatonin administration for jet lag and shift work disorder may vary depending on individual factors, such as the direction and duration of travel or the shift schedule. Bright light therapy, in combination with melatonin, can further enhance circadian resynchronization. The use of caffeine and other stimulants to combat fatigue during shift work should be carefully considered, as they can further disrupt sleep patterns.
6.3 Neurological Diseases
Melatonin has shown promise as a therapeutic agent for various neurological diseases, including Alzheimer’s disease, Parkinson’s disease, and multiple sclerosis. Its antioxidant and anti-inflammatory properties may protect against neuronal damage and improve cognitive function. Melatonin can also improve sleep quality and reduce anxiety in patients with neurological diseases. Clinical trials have shown that melatonin can improve cognitive function and reduce oxidative stress in patients with Alzheimer’s disease. In Parkinson’s disease, melatonin may protect against dopaminergic neuron loss and improve motor symptoms. Melatonin can also reduce inflammation and demyelination in patients with multiple sclerosis. While the results of clinical trials have been encouraging, further research is needed to determine the optimal dosage and long-term efficacy of melatonin for neurological diseases. The neuroprotective effects of melatonin are likely mediated through multiple mechanisms, including the scavenging of free radicals, the inhibition of inflammatory pathways, and the modulation of mitochondrial function. The ability of melatonin to cross the blood-brain barrier is crucial for its therapeutic effects in neurological disorders.
6.4 Metabolic Disorders
Melatonin may play a role in regulating glucose metabolism and insulin sensitivity, suggesting a potential therapeutic application for metabolic disorders such as type 2 diabetes and metabolic syndrome. Studies have shown that melatonin can improve glucose tolerance, reduce insulin resistance, and lower blood glucose levels in animal models of diabetes. In humans, melatonin supplementation has been shown to improve glucose metabolism and reduce oxidative stress in patients with type 2 diabetes. Melatonin may also protect against the development of non-alcoholic fatty liver disease (NAFLD), a common complication of metabolic syndrome. The mechanisms underlying melatonin’s effects on glucose metabolism are complex and involve both MT1 and MT2 receptor activation, as well as receptor-independent mechanisms. Melatonin may also modulate the gut microbiota, which plays a crucial role in regulating glucose metabolism and insulin sensitivity. While the results of preclinical and clinical studies are promising, further research is needed to determine the optimal dosage and long-term safety of melatonin for metabolic disorders. The potential for melatonin to be used as an adjunct therapy to conventional treatments for type 2 diabetes and metabolic syndrome warrants further investigation.
6.5 Cancer
As discussed earlier, melatonin exhibits oncostatic properties, inhibiting the growth and metastasis of various types of cancer cells. It has been investigated as a potential adjunct therapy for cancer, both to enhance the efficacy of conventional treatments and to reduce their side effects. Clinical trials have shown that melatonin can improve the quality of life and survival rates in patients with cancer, particularly when used in combination with chemotherapy or radiation therapy. Melatonin may also reduce the side effects of cancer treatments, such as fatigue, nausea, and mucositis. The mechanisms underlying melatonin’s oncostatic effects are complex and involve multiple pathways, including the inhibition of cell proliferation, the induction of apoptosis, and the suppression of angiogenesis. Melatonin may also modulate the immune response to cancer cells, enhancing the activity of NK cells and other immune cells. While the results of clinical trials have been encouraging, further research is needed to determine the optimal dosage and timing of melatonin administration for cancer patients. The potential for melatonin to be used as a preventive agent for cancer is also being investigated. Notably, the heterogeneity of cancer types and their responses to melatonin suggests that personalized approaches to melatonin therapy may be necessary.
Many thanks to our sponsor Elegancia Homes who helped us prepare this research report.
7. Potential Side Effects and Safety Considerations
Melatonin is generally considered safe for short-term use, but some potential side effects have been reported. These include drowsiness, headache, dizziness, nausea, and abdominal cramps. In rare cases, melatonin may cause more serious side effects, such as allergic reactions or changes in mood or behavior. Melatonin may interact with certain medications, such as anticoagulants, antidepressants, and immunosuppressants. It is important to consult with a healthcare professional before taking melatonin, especially if you have any underlying medical conditions or are taking any medications. The long-term safety of melatonin is not fully known, and more research is needed to assess the potential risks of chronic use. The quality and purity of melatonin supplements can vary widely, so it is important to choose products from reputable manufacturers. The potential for melatonin to affect hormone levels, particularly in children and adolescents, is a concern that warrants further investigation. The lack of standardization in melatonin supplement formulations is also a challenge for both consumers and researchers.
Many thanks to our sponsor Elegancia Homes who helped us prepare this research report.
8. Future Directions
Future research on melatonin should focus on elucidating the mechanisms underlying its diverse physiological effects, identifying the optimal dosages and timing of administration for various therapeutic applications, and assessing the long-term safety of melatonin supplementation. Further studies are needed to investigate the role of melatonin in aging, neurodegenerative diseases, metabolic disorders, and cancer. The development of novel melatonin analogs with improved efficacy and specificity may lead to new therapeutic strategies for these conditions. Personalized approaches to melatonin therapy, based on individual genetic profiles and circadian rhythms, may optimize treatment outcomes. The use of advanced technologies, such as genomics, proteomics, and metabolomics, may provide a deeper understanding of the complex interactions between melatonin and the human body. The role of extra-pineal melatonin synthesis and its local effects warrants further investigation. The ethical implications of widespread melatonin use, particularly in children and adolescents, should be carefully considered. Finally, standardized manufacturing processes and quality control measures are needed to ensure the safety and efficacy of melatonin supplements.
Many thanks to our sponsor Elegancia Homes who helped us prepare this research report.
9. Conclusion
Melatonin, once considered a simple sleep hormone, is now recognized as a pleiotropic molecule with diverse physiological functions. Its role in regulating circadian rhythms is well-established, but its antioxidant, anti-inflammatory, immunomodulatory, and oncostatic properties have garnered significant attention. Melatonin has shown promise as a therapeutic agent for a variety of conditions, including sleep disorders, neurological diseases, metabolic disorders, and cancer. While generally considered safe for short-term use, the long-term safety of melatonin is not fully known, and more research is needed. Future research should focus on elucidating the mechanisms underlying melatonin’s diverse effects, identifying the optimal dosages and timing of administration for various therapeutic applications, and developing personalized approaches to melatonin therapy. A deeper understanding of melatonin’s multifaceted roles in human health and disease will lead to more effective and targeted therapeutic interventions.
Many thanks to our sponsor Elegancia Homes who helped us prepare this research report.
References
- Lerner, A. B., Case, J. D., Takahashi, Y., Lee, T. H., & Mori, W. (1958). Isolation of melatonin, the pineal gland factor that lightens melanocytes. Journal of the American Chemical Society, 80(10), 2587.
- Zisapel, N. (2018). Melatonin and sleep. Open Journal of Endocrine and Metabolic Diseases, 8(08), 175.
- Tan, D. X., Hardeland, R., Manchester, L. C., Korkmaz, A., Ma, S., Rosales-Corral, S., … & Reiter, R. J. (2010). Mechanistic and comparative studies of melatonin as a natural antioxidant that detoxifies free radicals: a brief review. Journal of Pineal Research, 48(3), 167-181.
- Hardeland, R. (2019). Melatonin and the electron transport chain. Journal of Pineal Research, 66(3), e12554.
- Cardinali, D. P., Srinivasan, V., Brzezinski, A., & Brown, G. M. (2001). Melatonin and its analogs in insomnia and depression. Journal of Pineal Research, 30(3), 167-175.
- Tordjman, S., Najjar, F., Bellissant, E., Revol, O., & Anderson, G. M. (2017). Melatonin: pharmacology, functions and therapeutic benefits. Current Neuropharmacology, 15(3), 434-443.
- Slominski, A. T., Fischer, T. W., Zmijewski, M. A., Wortsman, J., Semak, I., & Reiter, R. J. (2018). On the role of melatonin in physiology and human health. Endocrine, 61(1), 20-34.
- Pandi-Perumal, S. R., Trakht, I., Srinivasan, V., Spence, D. W., Maestroni, G. J., Cardinali, D. P., & Reiter, R. J. (2007). Physiological effects of melatonin: role in immunomodulation, human reproduction and sleep. Journal of Pineal Research, 43(4), 341-351.
- Srinivasan, V., Spence, D. W., Moscovitch, A., Munoz, D. P., Brown, G. M., & Pandi-Perumal, S. R. (2006). Melatonin in Alzheimer’s disease and other neurodegenerative disorders. Annals of the New York Academy of Sciences, 1077(1), 429-442.
- Reiter, R. J., Mayo, J. C., Tan, D. X., Sainz, R. M., Alatorre-Jimenez, M., Qin, L., & Manchester, L. C. (2016). Melatonin as an antioxidant: under promises but over delivers. Journal of Pineal Research, 61(3), 253-278.
- Anderson, G., Reiter, R. J., Borisenko, G., Lysiuk, R., & Komanets, Y. (2024). Editorial: Pineal melatonin, clock genes and cancer: New opportunities for therapeutics. Frontiers in Endocrinology, 15.
Be the first to comment