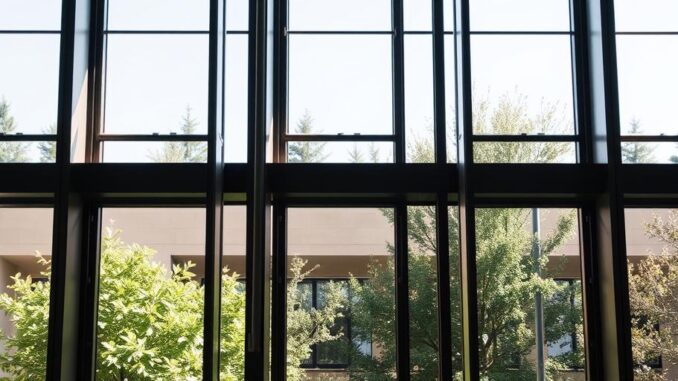
Optimizing Fenestration Design for Natural Light and Energy Performance: A Comprehensive Review
Abstract
Fenestration systems, encompassing windows, skylights, and other glazed openings, are critical architectural elements that significantly influence both the quantity and quality of natural light within buildings. Furthermore, they play a crucial role in regulating energy consumption through solar heat gain, heat loss, and the reduction of artificial lighting needs. This review comprehensively examines the intricate relationship between fenestration design and its impact on natural light optimization and overall energy performance. We explore various window types, glazing options, material properties, and advanced technologies, analyzing their individual and combined effects on daylighting metrics, thermal comfort, and building energy loads. Special attention is given to the influence of orientation, shading strategies, and integrated design approaches in achieving optimal fenestration solutions. Ultimately, this review aims to provide a robust framework for architects, engineers, and building designers to make informed decisions regarding fenestration design, leading to more sustainable, energy-efficient, and occupant-friendly built environments.
1. Introduction
Fenestration, derived from the Latin word ‘fenestra’ meaning window, represents a fundamental aspect of architectural design and building performance. Beyond its primary function of providing views and ventilation, fenestration plays a pivotal role in admitting natural light, which has been shown to improve occupant well-being, productivity, and overall satisfaction [1, 2]. However, uncontrolled solar heat gain through windows can significantly increase cooling loads, while excessive heat loss during colder months can escalate heating demands. Therefore, the design and selection of fenestration systems require a holistic approach, considering the interplay between daylighting, thermal performance, and energy efficiency. This review addresses the critical considerations for optimizing fenestration design, encompassing window types, glazing technologies, material selection, orientation strategies, shading mechanisms, and the integration of smart technologies.
2. Window Types and Their Impact on Daylighting
The selection of an appropriate window type is crucial for maximizing daylight penetration while minimizing undesirable effects such as glare and excessive solar heat gain. Different window types offer varying levels of control over airflow, aesthetics, and daylight distribution.
-
Casement Windows: Hinged on one side, casement windows swing outward, offering excellent ventilation and unobstructed views. Their design allows for a wide opening, maximizing daylight penetration, especially when strategically placed. However, their outward swing can be problematic in high-traffic areas or narrow spaces.
-
Sliding Windows: Consisting of two or more sashes that slide horizontally, sliding windows are easy to operate and offer good ventilation. While generally less airtight than casement windows, advancements in weather stripping have improved their energy performance. Their simpler design can be more cost-effective, but they may not provide the same level of unobstructed views as casement windows.
-
Awning Windows: Hinged at the top, awning windows swing outward and upward, providing ventilation even during light rain. Their design directs air inward from the top, offering a unique airflow pattern. Awning windows are often used in combination with other window types to enhance ventilation and daylighting in specific areas.
-
Fixed Windows: Also known as picture windows, fixed windows do not open and are primarily used for providing views and natural light. They offer excellent energy performance due to their airtight construction. Fixed windows are often incorporated into larger fenestration systems to maximize daylight penetration in areas where ventilation is not a primary concern.
-
Skylights: Installed on roofs, skylights offer a unique opportunity to introduce daylight from above, potentially providing a more even distribution of light throughout a space. However, skylights can also be a significant source of solar heat gain, particularly during summer months. Careful consideration must be given to glazing type, shading strategies, and orientation to mitigate these effects. Tubular daylighting devices (TDDs) are an alternative approach, channeling sunlight through reflective tubes to deliver daylight to interior spaces with minimal heat gain [3].
Choosing the appropriate window type depends on a multifaceted evaluation considering site conditions, orientation, desired ventilation, aesthetic goals, and budget constraints.
3. Glazing Options and Energy Efficiency
The glazing material constitutes a significant component of a window’s overall energy performance. The choice of glazing significantly impacts solar heat gain, heat loss, and the transmission of visible light. Numerous glazing options are available, each with unique properties and performance characteristics.
-
Single-Pane Glass: The most basic type of glazing, single-pane glass offers minimal insulation and is generally not recommended for energy-efficient buildings. It provides little resistance to heat transfer, leading to significant energy losses during colder months and excessive solar heat gain during warmer months.
-
Double-Pane Glass: Consisting of two panes of glass separated by an air or gas-filled space, double-pane glass offers significantly improved insulation compared to single-pane glass. The air or gas acts as a thermal barrier, reducing heat transfer and improving energy efficiency. Argon and krypton are commonly used gases due to their low thermal conductivity.
-
Low-E Coatings: Low-emissivity (Low-E) coatings are thin, transparent coatings applied to the surface of glass to reduce radiant heat transfer. Low-E coatings can be tailored to control solar heat gain, allowing for more daylight transmission while minimizing the amount of infrared radiation that enters the building. Different Low-E coatings are available for different climates and orientations, optimizing performance based on specific needs.
-
Tinted Glass: Tinted glass is manufactured with pigments added to the glass mixture, reducing the amount of visible light and solar heat that passes through. Tinted glass is effective in reducing glare and solar heat gain, but it can also reduce the amount of natural light entering the building, potentially increasing the need for artificial lighting. The choice of tint should be carefully considered to balance glare control with daylighting requirements.
-
Reflective Glass: Reflective glass features a metallic coating that reflects a significant portion of solar radiation, reducing solar heat gain. Reflective glass is often used in commercial buildings to minimize cooling loads, but it can also create glare problems for surrounding buildings. Careful design and orientation are essential to mitigate these issues.
-
Self-Cleaning Glass: Self-cleaning glass features a special coating that uses sunlight and rainwater to break down organic dirt and grime, keeping the glass clean with minimal maintenance. This is achieved through photocatalysis and hydrophilicity. While offering convenience, self-cleaning glass does not necessarily contribute to improved energy performance.
-
Electrochromic Glass: Electrochromic glass, also known as smart glass, allows for dynamic control of light and heat transmission. The glass changes its opacity in response to an electrical signal, allowing occupants to adjust the amount of daylight and solar heat entering the building. Electrochromic glass can be integrated with automated control systems to optimize energy performance based on real-time conditions.
The energy efficiency of glazing is typically measured using the following metrics:
-
U-Factor: Measures the rate of heat transfer through the glazing. Lower U-factors indicate better insulation performance.
-
Solar Heat Gain Coefficient (SHGC): Measures the fraction of solar radiation that enters the building through the glazing. Lower SHGC values indicate better solar heat gain control.
-
Visible Transmittance (VT): Measures the percentage of visible light that passes through the glazing. Higher VT values indicate greater daylight transmission.
Selecting the appropriate glazing option requires careful consideration of these metrics, as well as the building’s location, orientation, and climate.
4. Optimal Window Placement and Orientation
The orientation of windows significantly impacts the amount of solar radiation and natural light that enters a building. Optimal window placement can maximize daylighting benefits while minimizing unwanted solar heat gain.
-
North-Facing Windows: North-facing windows receive diffuse, indirect sunlight, providing consistent, glare-free illumination throughout the day. They are ideal for spaces where consistent daylight is desired, such as art studios or offices. North-facing windows generally have lower solar heat gain than windows facing other orientations, reducing cooling loads.
-
South-Facing Windows: South-facing windows receive direct sunlight during the winter months, providing passive solar heating. In the summer, however, they can be a major source of solar heat gain. Overhangs, awnings, and other shading devices are essential for controlling solar heat gain through south-facing windows. Proper design of these shading devices can allow for solar heat gain during the winter while blocking it during the summer [4].
-
East-Facing Windows: East-facing windows receive direct sunlight in the morning, providing natural light and warmth. However, they can also cause glare and overheating during the morning hours. Shading devices are recommended for east-facing windows, particularly in climates with hot summers.
-
West-Facing Windows: West-facing windows receive direct sunlight in the afternoon, often leading to excessive solar heat gain and glare. West-facing windows are generally the most challenging to manage in terms of solar control. External shading devices, such as trees or louvers, are particularly effective in blocking afternoon sun. The heat gain can be significant and contributes to discomfort and increased cooling loads.
Effective window placement also involves considering the depth of the room and the desired level of daylight penetration. Deeper rooms require larger windows or skylights to provide adequate daylighting to the interior spaces. Light shelves can be used to reflect daylight deeper into the room, improving the distribution of light [5].
5. Window Materials and Their Properties
The choice of window frame material significantly impacts the overall performance, aesthetics, and durability of the window. Different materials offer varying levels of insulation, maintenance requirements, and cost.
-
Wood: Wood frames offer excellent insulation and a natural aesthetic. However, wood requires regular maintenance, such as painting or staining, to prevent rot and decay. Wood frames can also be susceptible to moisture damage and insect infestation. High-quality wood frames can be very expensive.
-
Vinyl: Vinyl frames are low-maintenance, energy-efficient, and relatively inexpensive. Vinyl is resistant to moisture damage and insect infestation. However, vinyl frames can be less aesthetically pleasing than wood frames, and they may not be as durable in extreme climates. Some older vinyl frames are also prone to fading and cracking over time.
-
Aluminum: Aluminum frames are strong, durable, and low-maintenance. However, aluminum is a highly conductive material, meaning it transfers heat readily. Aluminum frames can be a significant source of heat loss or heat gain, particularly in extreme climates. Thermally broken aluminum frames, which incorporate a non-conductive barrier between the interior and exterior surfaces, can significantly improve their energy performance.
-
Fiberglass: Fiberglass frames offer a good balance of strength, durability, and energy efficiency. Fiberglass is resistant to moisture damage and insect infestation, and it has a low thermal expansion rate, making it less prone to cracking or warping. Fiberglass frames can be more expensive than vinyl frames but offer superior performance in some applications.
-
Composite: Composite frames are made from a combination of materials, such as wood fibers and polymers. Composite frames offer the aesthetic appeal of wood with the low-maintenance benefits of vinyl. They are resistant to moisture damage and insect infestation, and they offer good insulation performance. Composite frames can be a more expensive option than vinyl or aluminum frames.
The selection of window frame material should be based on a careful evaluation of factors such as climate, budget, aesthetic preferences, and maintenance requirements. Life cycle cost analysis can be used to compare the long-term costs of different materials, considering factors such as initial cost, energy savings, and maintenance expenses.
6. Shading Strategies and Daylighting Control
Effective shading strategies are essential for controlling solar heat gain and glare while maximizing daylight penetration. Shading devices can be external or internal, fixed or adjustable.
-
Overhangs and Awnings: Overhangs and awnings are fixed external shading devices that block direct sunlight from entering the building. The effectiveness of overhangs and awnings depends on their size, orientation, and the angle of the sun. Overhangs are particularly effective for south-facing windows, while awnings can be used to shade east- and west-facing windows.
-
Louvers: Louvers are adjustable or fixed slats that can be used to control the amount of sunlight entering the building. Louvers can be oriented horizontally or vertically, and they can be adjusted to block direct sunlight while allowing for ventilation. Louvers are particularly effective for shading east- and west-facing windows.
-
Blinds and Shades: Blinds and shades are internal shading devices that can be adjusted to control the amount of sunlight entering the building. Blinds are typically made of horizontal or vertical slats that can be tilted to block or redirect sunlight. Shades are typically made of fabric or other materials that can be raised or lowered to control the amount of light entering the room. Roller shades, cellular shades, and Roman shades are popular options. The reflective properties of the blind or shade material impacts the quality of light that is reflected into the room.
-
Trees and Landscaping: Trees and landscaping can provide effective shading, particularly for east- and west-facing windows. Deciduous trees are particularly useful, as they provide shade during the summer months and allow sunlight to penetrate during the winter months. Careful selection and placement of trees are essential to maximize their shading benefits.
-
Light Shelves: Light shelves are horizontal surfaces placed above windows to reflect daylight deeper into the room. Light shelves can improve the distribution of light and reduce the need for artificial lighting. Light shelves are particularly effective in rooms with high ceilings.
7. Smart Window Technologies
Emerging smart window technologies offer advanced control over daylighting, thermal performance, and energy efficiency.
-
Electrochromic Windows: As mentioned previously, electrochromic windows allow for dynamic control of light and heat transmission. They can be integrated with automated control systems to optimize energy performance based on real-time conditions. The ability to control the tint or opacity of the window dynamically can save significant energy.
-
Automated Shading Systems: Automated shading systems use sensors and controls to automatically adjust blinds, shades, or louvers based on the position of the sun, the time of day, and the occupancy of the building. Automated shading systems can optimize daylighting and reduce solar heat gain, leading to significant energy savings.
-
Building Integrated Photovoltaics (BIPV): BIPV systems integrate photovoltaic cells into the building envelope, generating electricity from sunlight. BIPV can be used to offset the building’s energy consumption and reduce its reliance on fossil fuels. BIPV windows can be designed to provide shading and reduce solar heat gain, while also generating electricity.
-
Aerogel Glazing: Aerogel is a highly porous material with exceptional thermal insulation properties. Aerogel glazing can significantly reduce heat transfer through windows, improving energy efficiency. Aerogel is currently expensive to manufacture, but ongoing research is focused on reducing costs and improving its performance.
8. Integrated Design Approach
Optimizing fenestration design requires an integrated design approach, considering all aspects of the building, including its orientation, climate, occupancy, and energy goals. An integrated design approach involves collaboration among architects, engineers, and building owners to develop a holistic fenestration solution that meets the building’s specific needs.
Key elements of an integrated design approach include:
-
Early consideration of fenestration: Fenestration should be considered early in the design process, allowing for optimization of window placement and orientation.
-
Use of simulation tools: Simulation tools can be used to model the daylighting and energy performance of different fenestration options.
-
Collaboration among disciplines: Architects, engineers, and building owners should work together to develop a holistic fenestration solution.
-
Life cycle cost analysis: Life cycle cost analysis can be used to compare the long-term costs of different fenestration options.
9. Conclusion
Fenestration plays a critical role in optimizing natural light, regulating energy consumption, and enhancing occupant comfort. This review has explored various aspects of fenestration design, including window types, glazing options, material properties, orientation strategies, shading mechanisms, and smart technologies. By carefully considering these factors and adopting an integrated design approach, architects, engineers, and building designers can create fenestration solutions that are both energy-efficient and aesthetically pleasing. Future research should focus on developing more advanced glazing materials, improving the performance of smart window technologies, and refining simulation tools to better predict the daylighting and energy performance of buildings. The implementation of these strategies is crucial for creating sustainable, high-performance buildings that minimize environmental impact and enhance the well-being of occupants.
References
[1] Edwards, L., & Torcellini, P. (2002). A literature review of the effects of natural light on building occupants. National Renewable Energy Laboratory (NREL).
[2] Boyce, P. R. (2003). Human factors in lighting. CRC press.
[3] Boubekri, M., Cheung, I. N. L., Reid, K. J., Wang, C. H., & Zee, P. C. (2014). Impact of windows and daylight on sleep quality and mood: A review. Journal of clinical sleep medicine : JCSM : official publication of the American Academy of Sleep Medicine, 10(1), 17–26.
[4] Moore, F. (2015). Environmental control systems: Heating, cooling, lighting. McGraw-Hill Education.
[5] Reinhart, C. F., & Walkenhorst, O. (2001). Dynamic RADIANCE-based daylight simulations for complex fenestration systems. Energy and Buildings, 33(7), 683-697.
The discussion on integrating BIPV systems into fenestration design is particularly interesting. Has anyone explored the long-term cost-effectiveness, considering both energy generation and potential maintenance challenges associated with these integrated systems?
Considering the impact of window orientation, how might predictive modeling be further refined to account for localized microclimates and urban density when optimizing fenestration design?
Considering the impact of window materials, what advancements are being made in bio-based or recycled composites to improve sustainability and reduce the embodied carbon footprint of fenestration systems?