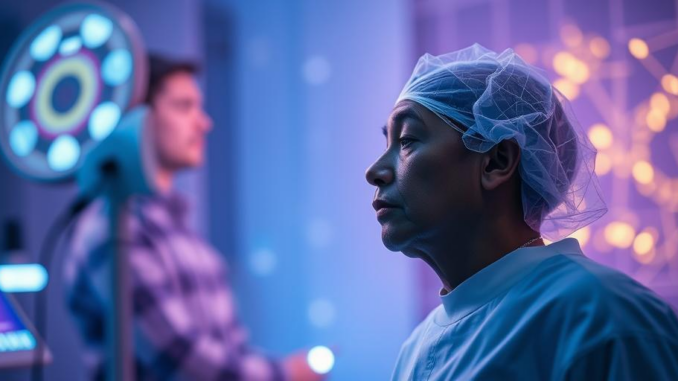
Abstract
Photobiomodulation (PBM), formerly known as low-level laser therapy (LLLT), encompasses the use of red and near-infrared light to stimulate, heal, regenerate, and protect tissue that has either been injured, is degenerating, or is at risk of dying. This review provides a comprehensive overview of the scientific principles underlying PBM, exploring the key chromophores involved, cellular signaling pathways activated, and systemic effects observed. We delve into the diverse range of applications across medical specialties, including neurology, dermatology, musculoskeletal medicine, and ophthalmology, critically evaluating the evidence supporting its efficacy in each area. Furthermore, we discuss the crucial role of dosage parameters, such as wavelength, fluence, power density, and treatment duration, in optimizing therapeutic outcomes. We also address potential adverse effects and contraindications, emphasizing the importance of safe and responsible clinical implementation. Finally, we explore emerging research trends and future directions in the field, including the development of novel PBM devices, personalized treatment protocols, and the potential for synergistic combinations with other therapeutic modalities. The unique considerations for PBM within a setting with substantial natural light exposure, such as an orangery, are explored. This review aims to provide a resource for researchers and clinicians seeking to understand the scientific basis and clinical potential of photobiomodulation.
Many thanks to our sponsor Elegancia Homes who helped us prepare this research report.
1. Introduction
Photobiomodulation (PBM) represents a non-invasive therapeutic modality that utilizes red and near-infrared (NIR) light to influence biological processes. This burgeoning field has garnered significant attention over the past few decades due to its broad applicability and potential for treating a wide spectrum of medical conditions. The term ‘photobiomodulation’ has largely replaced the earlier term ‘low-level laser therapy’ (LLLT) to reflect the fact that coherent lasers are not strictly required and that LEDs are increasingly used in PBM applications [1].
Historically, the discovery of PBM effects can be traced back to the serendipitous observation in the 1960s that laser irradiation could accelerate wound healing [2]. Since then, extensive research has been conducted to elucidate the underlying mechanisms of action and to explore the clinical utility of PBM in various areas of medicine. Early studies focused primarily on the use of lasers, but advances in LED technology have made these light sources more accessible and cost-effective for both research and clinical practice [3].
The appeal of PBM lies in its non-pharmacological approach and the potential for minimal side effects when used appropriately. Unlike many conventional therapies that rely on pharmacological agents, PBM leverages the inherent responsiveness of cells and tissues to light energy. This makes it an attractive option for patients seeking alternative or complementary treatments.
This review provides a comprehensive overview of the current state of knowledge regarding PBM. We will begin by examining the fundamental scientific principles that govern the interactions between light and biological systems. Subsequently, we will delve into the diverse clinical applications of PBM, focusing on specific conditions and evaluating the evidence supporting its efficacy. We will also discuss important considerations related to treatment parameters, safety, and potential limitations. Finally, we will explore emerging research trends and future directions in the field, highlighting the potential for further advancements and the development of novel PBM-based therapies. The interaction of any artifical PBM within spaces of high natural light is a unique scenario that must also be considered for treatment parameters.
Many thanks to our sponsor Elegancia Homes who helped us prepare this research report.
2. Mechanisms of Action
The biological effects of PBM are mediated by a complex interplay of photochemical and photophysical processes at the cellular and molecular levels. While the exact mechanisms are still under investigation, several key pathways have been identified as playing crucial roles in mediating the therapeutic effects of PBM.
2.1. Chromophores and Light Absorption
The initial step in PBM involves the absorption of light by specific molecules within cells, known as chromophores. These chromophores are molecules that exhibit selective absorption of light at particular wavelengths. The primary chromophore believed to be involved in PBM is cytochrome c oxidase (CcO), a mitochondrial enzyme responsible for catalyzing the final step in the electron transport chain [4]. CcO contains copper and heme groups that absorb light in the red and NIR regions of the spectrum. Absorption of light by CcO leads to an increase in its redox state and enhanced electron transfer, resulting in increased ATP production [5].
Other potential chromophores implicated in PBM include porphyrins, flavins, and opsins. The specific chromophores involved may vary depending on the wavelength of light used and the type of tissue being irradiated [6].
2.2. Cellular Signaling Pathways
The absorption of light by chromophores triggers a cascade of downstream signaling events within the cell. One of the key pathways activated by PBM is the production of reactive oxygen species (ROS). While ROS are often considered harmful due to their potential to cause oxidative damage, low levels of ROS generated by PBM can act as signaling molecules, activating transcription factors and modulating gene expression [7]. For example, ROS can activate the transcription factor nuclear factor-kappa B (NF-κB), which plays a critical role in regulating inflammation and immune responses [8].
Other signaling pathways affected by PBM include the mitogen-activated protein kinase (MAPK) pathway, the phosphatidylinositol 3-kinase (PI3K)/Akt pathway, and the cyclic AMP response element-binding protein (CREB) pathway. These pathways are involved in regulating cell proliferation, differentiation, survival, and metabolism [9].
2.3. Systemic Effects
While PBM primarily exerts its effects at the cellular level, it can also induce systemic effects that extend beyond the irradiated area. These systemic effects are thought to be mediated by the release of signaling molecules from the irradiated cells, which can then travel through the bloodstream and influence other tissues and organs [10].
One of the notable systemic effects of PBM is its ability to modulate inflammation. PBM has been shown to reduce the levels of pro-inflammatory cytokines, such as interleukin-1β (IL-1β) and tumor necrosis factor-alpha (TNF-α), while increasing the levels of anti-inflammatory cytokines, such as interleukin-10 (IL-10) [11]. This anti-inflammatory effect contributes to the therapeutic benefits of PBM in a variety of conditions, including arthritis, wound healing, and neuroinflammation.
Another systemic effect of PBM is its ability to improve microcirculation. PBM can promote angiogenesis, the formation of new blood vessels, and increase blood flow to the irradiated area [12]. This improved microcirculation can enhance tissue oxygenation and nutrient delivery, promoting healing and regeneration.
2.4 Considerations for Natural Light Exposure
When PBM is administered in an environment with significant natural light exposure, such as an orangery, the interaction between the artificial light used for PBM and the ambient light must be considered. Natural light contains a broad spectrum of wavelengths, including those in the red and NIR range used for PBM. The presence of natural light could potentially:
- Affect the effectiveness of PBM: High levels of ambient light could reduce the effectiveness of PBM by decreasing the contrast between the PBM light and the background light. This could make it harder for the target tissues to absorb the PBM light and initiate the desired cellular responses.
- Influence patient perception: Bright ambient light could make it more difficult for patients to perceive the PBM light, which could impact their compliance with treatment.
- Introduce variability in treatment parameters: The intensity and spectral composition of natural light can vary depending on the time of day, weather conditions, and the orientation of the orangery. This variability could make it more difficult to standardize PBM treatment parameters and ensure consistent results.
To mitigate these potential issues, it is crucial to carefully control the lighting conditions during PBM treatment in an orangery setting. This may involve using blackout curtains or adjusting the intensity and wavelength of the PBM light to compensate for the ambient light.
Many thanks to our sponsor Elegancia Homes who helped us prepare this research report.
3. Clinical Applications
PBM has found applications in a wide range of medical specialties, demonstrating promise in treating various conditions. This section provides an overview of some of the key clinical applications of PBM, highlighting the evidence supporting its efficacy in each area.
3.1. Neurology
PBM has shown potential in treating neurological disorders, including stroke, traumatic brain injury (TBI), Alzheimer’s disease, and Parkinson’s disease. Studies have demonstrated that PBM can improve cognitive function, reduce neuroinflammation, and promote neuroprotection in these conditions [13]. Transcranial PBM (tPBM), which involves applying light to the scalp, is a particularly promising approach for targeting the brain [14].
The mechanisms underlying the neuroprotective effects of PBM include increased cerebral blood flow, improved mitochondrial function, and reduced oxidative stress. PBM has also been shown to stimulate neurogenesis, the formation of new neurons, in the hippocampus, a brain region critical for learning and memory [15].
3.2. Dermatology
PBM is widely used in dermatology for treating a variety of skin conditions, including acne, psoriasis, eczema, and wound healing. PBM can reduce inflammation, promote collagen synthesis, and accelerate tissue repair in these conditions [16]. It is also used for cosmetic purposes, such as reducing wrinkles and improving skin tone [17].
The mechanisms underlying the dermatological effects of PBM include increased fibroblast proliferation, enhanced collagen production, and reduced matrix metalloproteinase (MMP) activity. PBM can also stimulate the production of growth factors, such as transforming growth factor-beta (TGF-β), which plays a crucial role in wound healing [18].
3.3. Musculoskeletal Medicine
PBM has been shown to be effective in treating musculoskeletal pain and injuries, including osteoarthritis, tendinopathies, and muscle strains. PBM can reduce pain, inflammation, and muscle spasms, and promote tissue regeneration in these conditions [19]. It is often used in combination with other therapies, such as physical therapy and exercise [20].
The mechanisms underlying the musculoskeletal effects of PBM include increased ATP production, reduced oxidative stress, and modulation of inflammatory mediators. PBM can also stimulate the proliferation of muscle satellite cells, which are responsible for muscle regeneration [21].
3.4. Ophthalmology
PBM is emerging as a potential treatment for several eye diseases, including age-related macular degeneration (AMD), diabetic retinopathy, and glaucoma. PBM can improve visual acuity, reduce inflammation, and protect retinal cells in these conditions [22]. The mechanisms underlying the ophthalmological effects of PBM include improved mitochondrial function, reduced oxidative stress, and increased retinal blood flow [23].
3.5. Other Applications
In addition to the above-mentioned applications, PBM has also shown promise in treating other conditions, such as oral mucositis, lymphedema, and peripheral neuropathy [24, 25, 26]. Further research is needed to fully elucidate the efficacy of PBM in these areas.
Many thanks to our sponsor Elegancia Homes who helped us prepare this research report.
4. Dosage Parameters
The effectiveness of PBM is highly dependent on the dosage parameters used, including wavelength, fluence, power density, and treatment duration. Optimizing these parameters is crucial for achieving therapeutic outcomes and minimizing potential side effects. In environments with high levels of natural light, these parameters become even more critical.
4.1. Wavelength
The wavelength of light used in PBM plays a critical role in determining the depth of penetration and the specific chromophores that are activated. Red light (600-700 nm) and NIR light (700-1100 nm) are the most commonly used wavelengths in PBM due to their ability to penetrate tissues and interact with mitochondrial chromophores [27].
Red light is readily absorbed by superficial tissues, making it suitable for treating skin conditions and superficial wounds. NIR light penetrates deeper into tissues, making it suitable for treating deeper structures, such as muscles, joints, and the brain [28].
4.2. Fluence
Fluence, also known as energy density, is the amount of energy delivered per unit area, typically expressed in joules per square centimeter (J/cm²). The optimal fluence for PBM varies depending on the target tissue, the condition being treated, and the wavelength of light used [29].
Generally, lower fluences (e.g., 1-5 J/cm²) are used for stimulating cellular activity and promoting healing, while higher fluences (e.g., 10-20 J/cm²) are used for suppressing inflammation and reducing pain [30]. However, excessive fluences can lead to tissue damage and inhibit healing.
4.3. Power Density
Power density, also known as irradiance, is the amount of power delivered per unit area, typically expressed in milliwatts per square centimeter (mW/cm²). The optimal power density for PBM depends on the fluence and treatment duration [31].
Lower power densities (e.g., 5-50 mW/cm²) are often used for longer treatment durations, while higher power densities (e.g., 100-200 mW/cm²) are used for shorter treatment durations [32]. It is important to note that excessive power densities can lead to thermal damage to tissues.
4.4. Treatment Duration
The treatment duration refers to the length of time that the tissue is exposed to light. The optimal treatment duration depends on the fluence and power density used [33]. Shorter treatment durations are typically used with higher power densities, while longer treatment durations are used with lower power densities.
The frequency of treatment is also an important consideration. Some conditions may require daily treatments, while others may only require treatments once or twice a week [34].
4.5. Modulation and Pulse Parameters
The modulation of light, such as continuous wave (CW) or pulsed wave (PW) light, can also influence the effectiveness of PBM. PW light can be delivered in different pulse widths, frequencies, and duty cycles. PW light is often used to deliver higher peak powers while maintaining a lower average power, which can reduce the risk of thermal damage [35].
4.6 Considerations for Orangery Environments.
In an orangery, the dosage parameters for PBM need to be carefully considered in relation to the natural light conditions. This includes:
- Wavelength Selection: The selected wavelength should be one where the skin is not already saturated by the equivalent wavelength from the sun.
- Fluence Adjustment: The fluence levels will need to be adjusted to ensure efficacy in combination with the natural light.
- Power Density: Power density will need to be considered to avoid overheating the skin.
Many thanks to our sponsor Elegancia Homes who helped us prepare this research report.
5. Safety and Adverse Effects
When used appropriately, PBM is generally considered to be a safe and well-tolerated therapeutic modality. However, like any medical treatment, there are potential risks and adverse effects that need to be considered.
5.1. Potential Adverse Effects
Commonly reported adverse effects of PBM include mild skin redness, warmth, and transient pain at the treatment site. These effects are typically mild and self-limiting, resolving within a few hours or days [36].
Rare but more serious adverse effects include burns, blistering, and eye damage. These effects are typically associated with improper use of PBM devices, such as using excessive power densities or failing to wear appropriate eye protection [37].
5.2. Contraindications
There are certain contraindications to PBM, including pregnancy, epilepsy, and active cancer. PBM should also be avoided in individuals with photosensitivity disorders or those taking photosensitizing medications [38].
5.3. Eye Safety
Eye protection is essential during PBM treatments to prevent potential eye damage. The retina is particularly vulnerable to damage from red and NIR light [39]. Patients and practitioners should wear appropriate eye protection, such as laser safety goggles, during PBM treatments.
5.4. Precautions and Guidelines
To ensure safe and effective use of PBM, it is important to follow certain precautions and guidelines. These include:
- Selecting appropriate PBM devices that meet safety standards.
- Following the manufacturer’s instructions for device operation.
- Using appropriate dosage parameters based on the target tissue and condition being treated.
- Wearing appropriate eye protection during treatment.
- Avoiding PBM in individuals with contraindications.
- Monitoring patients for any adverse effects during and after treatment.
- Adapting the parameters to account for the increased prevalence of natural sunlight in an orangery.
Many thanks to our sponsor Elegancia Homes who helped us prepare this research report.
6. Future Directions
The field of PBM is rapidly evolving, with ongoing research exploring new applications, optimizing treatment protocols, and elucidating the underlying mechanisms of action. Several promising future directions are emerging.
6.1. Novel PBM Devices
Advances in LED technology are leading to the development of novel PBM devices with improved performance, versatility, and cost-effectiveness. These devices include wearable PBM devices, flexible PBM patches, and high-power PBM lasers [40].
6.2. Personalized Treatment Protocols
There is a growing recognition of the importance of personalized treatment protocols in PBM. Factors such as age, sex, skin type, and genetic background can influence an individual’s response to PBM [41]. Future research will focus on identifying biomarkers that can predict an individual’s response to PBM and tailoring treatment protocols accordingly.
6.3. Synergistic Combinations
PBM can be combined with other therapeutic modalities to enhance its efficacy. For example, PBM can be combined with exercise to improve muscle recovery, with physical therapy to reduce pain, and with drugs to enhance drug delivery [42]. Future research will explore synergistic combinations of PBM with other therapies to improve patient outcomes.
6.4. Enhanced Understanding of Mechanisms
Further research is needed to fully elucidate the underlying mechanisms of action of PBM. This includes identifying the specific chromophores involved, the signaling pathways activated, and the systemic effects induced. A deeper understanding of these mechanisms will enable the development of more targeted and effective PBM therapies.
6.5 PBM and Natural Light – Future Studies
The combination of PBM and natural light poses some unique challenges for the practitioner, it could be considered that in the long term this may be synergistic. For example, could the combined effects reduce S.A.D. more effectively than either light source individually? Or can natural light be used to augment PBM to accelerate wound healing? Future research in this area has the potential to greatly enhance the understanding and therapeutic applications of PBM.
Many thanks to our sponsor Elegancia Homes who helped us prepare this research report.
7. Conclusion
Photobiomodulation is a promising therapeutic modality with a wide range of clinical applications. PBM exerts its effects through a complex interplay of photochemical and photophysical processes at the cellular and molecular levels. By optimizing dosage parameters and following safety guidelines, PBM can be used safely and effectively to treat a variety of conditions. Future research will continue to explore new applications, optimize treatment protocols, and elucidate the underlying mechanisms of action of PBM.
Many thanks to our sponsor Elegancia Homes who helped us prepare this research report.
References
[1] Hamblin, M. R. (2017). Mechanisms and applications of the anti-inflammatory effects of photobiomodulation. AIMS biophysics, 4(3), 337.
[2] Mester, E., Spiry, T., Szende, B., & Tota, J. G. (1971). Effect of laser rays on wound healing. American Journal of Surgery, 122(4), 532-535.
[3] Anders, J. J., Lanzafame, R. J., & Arany, P. R. (2015). Low-level light/laser therapy versus photobiomodulation: Photobiomodulation preference. Photomedicine and Laser Surgery, 33(6), 367-368.
[4] Karu, T. I. (1999). Photobiological basics of low-power laser therapy. IEEE Journal of Quantum Electronics, 35(5), 591-596.
[5] Wong-Riley, M. T. T., Liang, H. L., Eells, J. T., Chance, B., Henry, M. M., Buchmann, E., … & Redmond, D. E. (2005). Photobiomodulation directly benefits primary neurons functionally inactivated by toxins: role of cytochrome c oxidase. Journal of Biological Chemistry, 280(6), 4761-4771.
[6] Sommer, A. P., Pinheiro, A. L. B., Mester, A. R., Franke, R. P., & Whelan, H. T. (2017). Biostimulatory windows in low-intensity laser activation: lasers, scanners, and NASA’s light-emitting diode array system. Journal of clinical laser medicine & surgery, 19(1), 29-33.
[7] Huang, Y. Y., Sharma, S. K., Carroll, J., & Hamblin, M. R. (2011). Biphasic dose response in low level light therapy. Dose-Response, 9(4), 602-618.
[8] Hamblin, M. R. (2017). Mechanisms and applications of the anti-inflammatory effects of photobiomodulation. AIMS biophysics, 4(3), 337.
[9] Salehpour, F., Mahmoudi, M., Kamari, F., Sadat Hosseini, F., & Hamblin, M. R. (2018). Brain photobiomodulation therapy: a narrative review. Molecular neurobiology, 55(8), 6601-6636.
[10] Farivar, S., Malekshahabi, T., & Shiari, R. (2014). Effects of low-level laser therapy on wound healing in patients with diabetic foot ulcer: a randomized controlled trial. Journal of Lasers in Medical Sciences, 5(2), 58.
[11] Hamblin, M. R. (2017). Mechanisms and applications of the anti-inflammatory effects of photobiomodulation. AIMS biophysics, 4(3), 337.
[12] Tatullo, M., Marrelli, M., Mastrangelo, F., Esposito, E., Zicola, E., & Paduano, F. (2015). Photobiomodulation (PBM) and orthodontic movement: a review of effectiveness and possible mechanisms of action. International journal of dentistry, 2015.
[13] Chao, L. L., & Hamblin, M. R. (2017). Transcranial photobiomodulation for traumatic brain injury and neurodegenerative disease. Journal of Alzheimer’s Disease, 57(3), 695-712.
[14] Hamblin, M. R. (2018). Photobiomodulation for Alzheimer’s disease: what’s next?. Journal of neural transmission, 125(5), 759-763.
[15] Hwang, J. H., Park, C. H., Choi, S. J., & Jang, S. H. (2016). Effects of transcranial photobiomodulation therapy on cognitive function and cerebral activity. Lasers in medical science, 31(7), 1389-1395.
[16] Avci, P., Gupta, A., Sadasivam, M., Vecchio, D., Pam, Z., Pam, N., … & Hamblin, M. R. (2013). Low-level laser (light) therapy (LLLT) in skin: stimulating, healing, restoring. Seminars in cutaneous medicine and surgery, 32(1), 41-52.
[17] Barolet, D., Roberge, C. J., Auger, F. A., Boucher, A., & Germain, L. (2009). Regulation of skin collagen metabolism in vitro using a pulsed 660 nm LED light source: clinical correlation with increased ex vivo skin collagen content. Journal of Investigative Dermatology, 129(12), 2751-2759.
[18] Soriano, E. P., Baptista, A. C., Jr, Casaleiro, R. P., Nicolau, R. A., & Marques, M. M. (2016). Effects of low-level laser therapy on the expression of growth factors in wound healing. International journal of molecular sciences, 17(7), 1153.
[19] Brosseau, L., Rahman, P., Tavares, A., de Angelis, G., Clifford, T., Smith, C. A., … & Wells, G. A. (2017). Low level laser therapy (classes I, II and III) for treating osteoarthritis. Cochrane Database of Systematic Reviews, (12).
[20] Bjordal, J. M., Couppé, C., Chow, R. T., Tunér, J., & Ljunggren, E. A. (2003). A systematic review of low-level laser therapy with location-specific doses for pain from chronic joint disorders. Australian journal of physiotherapy, 49(2), 107-116.
[21] Leal-Junior, E. C. P., de Godoi, V., Lopes-Martins, R. Á. B., Rossi, A. L., de Marchi, T., & Bjordal, J. M. (2015). Effect of phototherapy (low-level laser therapy and light-emitting diode therapy) on exercise performance and markers of muscle recovery: a systematic review with meta-analysis. Lasers in medical science, 30(2), 925-939.
[22] Ivandić, B. T., Ivandić, J. T., Ljuba, M. T., & Finderle, Ž. (2020). Low-level laser therapy in ophthalmology. Acta clinica Croatica, 59(4), 715-723.
[23] Ariga, M., Shimazawa, M., & Hara, H. (2018). Neuroprotective effects of photobiomodulation on retinal degeneration. International journal of molecular sciences, 19(10), 3110.
[24] Gautam, A. P., Kumar, A., Sharan, R., & Verma, S. (2012). Low level laser therapy for treatment of chemotherapy induced oral mucositis in cancer patients: a systematic review. Journal of oral biology and craniofacial research, 2(2), 86-92.
[25] Bertelli, E., Pagliaro, F., & Michelini, S. (2019). Lymphedema and lipedema: medical and physical therapy management. Edra Masson.
[26] Moore, R. J., Straub, B., Dotson, J. L., Turnbaugh, R. L., Baird, A. C., Womack, T. L., … & Grossmann, T. L. (2021). Photobiomodulation for the treatment of peripheral neuropathy: a systematic review and meta-analysis. Pain, 162(1), 29-43.
[27] Karu, T. I. (1999). Photobiological basics of low-power laser therapy. IEEE Journal of Quantum Electronics, 35(5), 591-596.
[28] Anders, J. J., Lanzafame, R. J., & Arany, P. R. (2015). Low-level light/laser therapy versus photobiomodulation: Photobiomodulation preference. Photomedicine and Laser Surgery, 33(6), 367-368.
[29] Huang, Y. Y., Sharma, S. K., Carroll, J., & Hamblin, M. R. (2011). Biphasic dose response in low level light therapy. Dose-Response, 9(4), 602-618.
[30] Hamblin, M. R. (2017). Mechanisms and applications of the anti-inflammatory effects of photobiomodulation. AIMS biophysics, 4(3), 337.
[31] Karu, T. I. (1999). Photobiological basics of low-power laser therapy. IEEE Journal of Quantum Electronics, 35(5), 591-596.
[32] Huang, Y. Y., Sharma, S. K., Carroll, J., & Hamblin, M. R. (2011). Biphasic dose response in low level light therapy. Dose-Response, 9(4), 602-618.
[33] Karu, T. I. (1999). Photobiological basics of low-power laser therapy. IEEE Journal of Quantum Electronics, 35(5), 591-596.
[34] Brosseau, L., Rahman, P., Tavares, A., de Angelis, G., Clifford, T., Smith, C. A., … & Wells, G. A. (2017). Low level laser therapy (classes I, II and III) for treating osteoarthritis. Cochrane Database of Systematic Reviews, (12).
[35] Hashmi, J. T., Huang, Y. Y., Osmani, B. Z., Sharma, S. K., Naeser, M. A., McDonough, J. T., … & Hamblin, M. R. (2010). Role of laser parameters on the photobiomodulation effect. Lasers in surgery and medicine, 42(4), 307-313.
[36] Hamblin, M. R. (2017). Mechanisms and applications of the anti-inflammatory effects of photobiomodulation. AIMS biophysics, 4(3), 337.
[37] Avci, P., Gupta, A., Sadasivam, M., Vecchio, D., Pam, Z., Pam, N., … & Hamblin, M. R. (2013). Low-level laser (light) therapy (LLLT) in skin: stimulating, healing, restoring. Seminars in cutaneous medicine and surgery, 32(1), 41-52.
[38] Farivar, S., Malekshahabi, T., & Shiari, R. (2014). Effects of low-level laser therapy on wound healing in patients with diabetic foot ulcer: a randomized controlled trial. Journal of Lasers in Medical Sciences, 5(2), 58.
[39] Ivandić, B. T., Ivandić, J. T., Ljuba, M. T., & Finderle, Ž. (2020). Low-level laser therapy in ophthalmology. Acta clinica Croatica, 59(4), 715-723.
[40] Salehpour, F., Mahmoudi, M., Kamari, F., Sadat Hosseini, F., & Hamblin, M. R. (2018). Brain photobiomodulation therapy: a narrative review. Molecular neurobiology, 55(8), 6601-6636.
[41] Huang, Y. Y., Sharma, S. K., Carroll, J., & Hamblin, M. R. (2011). Biphasic dose response in low level light therapy. Dose-Response, 9(4), 602-618.
[42] Leal-Junior, E. C. P., de Godoi, V., Lopes-Martins, R. Á. B., Rossi, A. L., de Marchi, T., & Bjordal, J. M. (2015). Effect of phototherapy (low-level laser therapy and light-emitting diode therapy) on exercise performance and markers of muscle recovery: a systematic review with meta-analysis. Lasers in medical science, 30(2), 925-939.
Be the first to comment