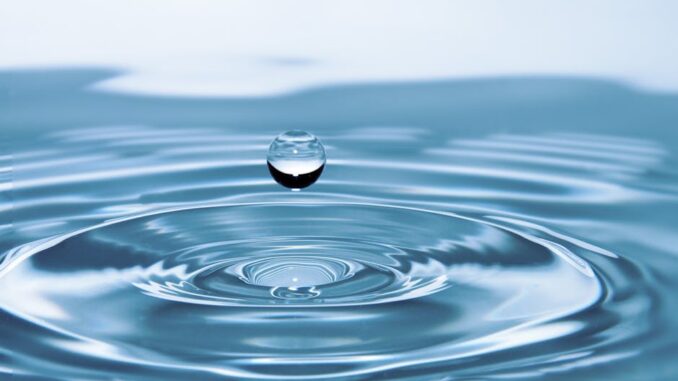
The Dynamic Interplay of Light, Water, and Nutrient Acquisition in Plant Physiology: Implications for Managed Environments
Abstract
This research report delves into the intricate physiological mechanisms governing light, water, and nutrient acquisition in plants, with a particular emphasis on the challenges and opportunities presented by managed environments such as orangeries. We explore the fundamental processes of photosynthesis, transpiration, and nutrient uptake, examining how these processes are interconnected and influenced by environmental factors. Furthermore, we analyze the adaptive strategies plants employ to optimize resource acquisition under varying conditions, considering the molecular and cellular mechanisms involved. The report highlights the significance of understanding these physiological principles for effective plant management, particularly in controlled settings where environmental parameters can be manipulated to enhance growth and productivity. We also discuss emerging research areas, including the role of plant hormones, signaling pathways, and the microbiome in regulating resource acquisition, and suggest avenues for future investigation to improve plant performance in both natural and managed ecosystems.
1. Introduction
Plants, as sessile organisms, face the constant challenge of acquiring essential resources – light, water, and nutrients – from their surrounding environment. Their survival and growth depend on the efficient capture and utilization of these resources, a process that is intricately regulated by complex physiological mechanisms. This report aims to provide a comprehensive overview of these mechanisms, focusing on the dynamic interplay between light, water, and nutrient acquisition, and their relevance to plant performance in managed environments.
Managed environments, such as orangeries, greenhouses, and controlled-environment agriculture systems, offer the potential to optimize plant growth by manipulating environmental factors. However, realizing this potential requires a deep understanding of plant physiology and the specific needs of different plant species. Understanding the interactions between light, water, and nutrient availability is crucial for optimizing plant growth and health in these environments. Incorrectly managing these factors can lead to reduced productivity, disease susceptibility, and even plant death.
This report will explore the key physiological processes involved in resource acquisition, including photosynthesis, transpiration, and nutrient uptake, and examine how these processes are interconnected and influenced by environmental factors. It will also discuss the adaptive strategies plants employ to optimize resource acquisition under varying conditions, and the molecular and cellular mechanisms involved. Furthermore, the report will highlight the significance of understanding these physiological principles for effective plant management, particularly in controlled settings where environmental parameters can be manipulated to enhance growth and productivity. We will also explore emerging research areas and suggest avenues for future investigation to improve plant performance in both natural and managed ecosystems.
2. Photosynthesis: Capturing Light Energy
Photosynthesis is the fundamental process by which plants convert light energy into chemical energy in the form of sugars. This process occurs in chloroplasts, organelles within plant cells that contain the pigment chlorophyll, which absorbs light energy. The process involves two main stages: the light-dependent reactions and the light-independent reactions (Calvin cycle).
The light-dependent reactions capture light energy and convert it into chemical energy in the form of ATP and NADPH. Chlorophyll molecules absorb light energy, which excites electrons. These electrons are then passed along an electron transport chain, generating a proton gradient across the thylakoid membrane. This proton gradient drives the synthesis of ATP through chemiosmosis. Meanwhile, water molecules are split, releasing electrons to replenish those lost by chlorophyll, and producing oxygen as a byproduct. The final step involves the reduction of NADP+ to NADPH.
The light-independent reactions, or Calvin cycle, use the ATP and NADPH produced during the light-dependent reactions to fix carbon dioxide from the atmosphere and convert it into glucose. This cycle involves a series of enzymatic reactions that ultimately regenerate the starting molecule, RuBP, allowing the cycle to continue. Factors affecting photosynthesis are light intensity, carbon dioxide concentration and temperature.
2.1 Light Quality and Intensity
The rate of photosynthesis is directly proportional to light intensity, up to a certain point. Beyond this point, the rate of photosynthesis plateaus as other factors, such as carbon dioxide availability, become limiting. Light quality, or the spectral composition of light, also influences photosynthesis. Chlorophyll absorbs light most efficiently in the red and blue regions of the spectrum. Therefore, plants grown under light sources that emit primarily in these regions, such as LED lights, can exhibit higher rates of photosynthesis than plants grown under light sources with a different spectral composition.
2.2 Carbon Dioxide Availability
Carbon dioxide is an essential substrate for the Calvin cycle. Therefore, the rate of photosynthesis is also dependent on carbon dioxide concentration. In many environments, carbon dioxide concentration can be a limiting factor for photosynthesis. This is particularly true in closed environments, such as greenhouses, where carbon dioxide can be depleted by plant uptake. Therefore, supplemental carbon dioxide can often increase the rate of photosynthesis and plant growth in these environments.
2.3 Temperature Effects
Temperature also influences the rate of photosynthesis. Photosynthesis is an enzymatic process, and the rate of enzymatic reactions is generally temperature-dependent. However, excessively high temperatures can denature enzymes and damage the photosynthetic machinery, inhibiting photosynthesis. Different plant species have different temperature optima for photosynthesis. In controlled environments, maintaining the optimal temperature range for photosynthesis can significantly enhance plant growth.
2.4 Photoacclimation
Plants can adjust their photosynthetic machinery in response to changes in light availability, a process known as photoacclimation. For example, plants grown in low-light environments tend to have higher levels of chlorophyll and larger light-harvesting complexes than plants grown in high-light environments. This allows them to capture more light energy under low-light conditions. Conversely, plants grown in high-light environments tend to have higher levels of antioxidants to protect against photo-oxidative damage.
3. Transpiration: The Water Highway
Transpiration is the process by which water is lost from plants through evaporation, primarily from the stomata, which are tiny pores on the leaf surface. While transpiration can seem like a disadvantage, as it requires plants to constantly replenish water, it is actually a crucial process for several reasons.
First, transpiration drives the movement of water and nutrients from the roots to the shoots. As water evaporates from the leaves, it creates a tension that pulls water up the xylem, the vascular tissue that transports water throughout the plant. This process, known as the cohesion-tension theory, is the primary mechanism by which water is transported in plants. This enables plants to absorb mineral nutrients from the soil.
Second, transpiration cools the leaves. As water evaporates, it absorbs heat energy, thereby lowering the temperature of the leaves. This is particularly important in hot environments, where excessive heat can damage plant tissues. In fact, this cooling effect is essential to prevent overheating in high radiation envionments.
3.1 Factors Influencing Transpiration
Several factors influence the rate of transpiration, including temperature, humidity, wind speed, and light intensity.
- Temperature: Higher temperatures increase the rate of evaporation, and therefore increase the rate of transpiration.
- Humidity: Lower humidity increases the rate of evaporation, and therefore increases the rate of transpiration.
- Wind Speed: Higher wind speed removes water vapor from the leaf surface, increasing the rate of evaporation and transpiration.
- Light Intensity: Higher light intensity can increase the rate of transpiration by increasing leaf temperature and by stimulating stomatal opening. Stomatal opening is controlled by guard cells, which surround the stomata. Guard cells respond to various environmental signals, including light, carbon dioxide concentration, and water stress.
3.2 Stomatal Regulation
The opening and closing of stomata is tightly regulated to balance the need for carbon dioxide uptake for photosynthesis with the need to conserve water. When water is plentiful, the stomata open to allow carbon dioxide to enter the leaves for photosynthesis. However, when water is scarce, the stomata close to prevent excessive water loss. This regulation is crucial for plant survival in drought conditions. The plant hormone abscisic acid (ABA) plays a key role in stomatal closure. Under water stress, ABA levels increase, triggering a signaling cascade that leads to the closing of stomata.
3.3 Adaptations to Reduce Transpiration
Plants have evolved various adaptations to reduce transpiration in arid environments. These adaptations include: reduced leaf size, thick cuticles, sunken stomata, and the presence of trichomes (hairs) on the leaf surface. These adaptations help to reduce water loss by decreasing the surface area for evaporation, increasing the resistance to water movement, and creating a boundary layer of humid air around the leaf.
4. Nutrient Acquisition: Feeding the Plant
Plants require a variety of essential nutrients for growth and development. These nutrients are typically divided into macronutrients and micronutrients. Macronutrients are required in relatively large amounts, while micronutrients are required in relatively small amounts. Macronutrients include nitrogen, phosphorus, potassium, calcium, magnesium, and sulfur. Micronutrients include iron, manganese, zinc, copper, boron, molybdenum, and chlorine.
4.1 Nutrient Uptake Mechanisms
Plants acquire nutrients from the soil through their roots. Nutrient uptake is an active process that requires energy. Plants use specialized transport proteins in their root cell membranes to transport nutrients against their concentration gradients. Nutrients can be absorbed via two main pathways: the apoplast pathway and the symplast pathway. In the apoplast pathway, nutrients move through the cell walls and intercellular spaces without entering the cells. In the symplast pathway, nutrients enter the cells and move through the cytoplasm via plasmodesmata, which are channels that connect adjacent plant cells. Once nutrients reach the vascular tissue in the root, they are transported throughout the plant in the xylem.
4.2 The Role of Mycorrhizae
Many plants form symbiotic relationships with fungi called mycorrhizae. Mycorrhizae enhance nutrient uptake by increasing the surface area of the root system. The fungal hyphae extend far beyond the root zone, accessing nutrients that would otherwise be unavailable to the plant. In exchange for nutrients, the plant provides the fungus with carbohydrates. Mycorrhizal associations are particularly important for the uptake of phosphorus, which is often immobile in the soil. There are two main types of mycorrhizae: ectomycorrhizae and endomycorrhizae. Ectomycorrhizae form a sheath around the root, while endomycorrhizae penetrate the root cells.
4.3 Nutrient Deficiency and Toxicity
Nutrient deficiencies can lead to various symptoms, depending on the nutrient that is lacking. For example, nitrogen deficiency can cause yellowing of the leaves (chlorosis), while phosphorus deficiency can cause stunted growth and purple coloration of the leaves. Nutrient toxicities can also be harmful to plants. For example, excessive levels of heavy metals can inhibit plant growth and development.
4.4 Nutrient Management in Managed Environments
In managed environments, nutrient availability can be carefully controlled. Growers can use fertilizers to provide plants with the nutrients they need. Different types of fertilizers are available, including organic fertilizers and inorganic fertilizers. Organic fertilizers are derived from natural sources, such as compost and manure, while inorganic fertilizers are synthesized chemically. The type of fertilizer used will depend on the specific needs of the plants and the growing conditions. In hydroponic systems, plants are grown in nutrient solutions without soil. This allows for precise control over nutrient availability.
5. Interconnectedness of Light, Water, and Nutrient Acquisition
The acquisition of light, water, and nutrients are not independent processes. They are intricately interconnected and influence each other in various ways. For example, light intensity affects the rate of photosynthesis, which in turn affects the demand for water and nutrients. Water availability affects the rate of transpiration, which in turn affects nutrient uptake. Nutrient availability affects the synthesis of chlorophyll, which in turn affects light capture. To understand the complex plant physiology, it is crucial to appreciate how all these processes are interconnected and regulate each other.
5.1 Light and Water
As discussed above, high light intensity often results in increased transpiration rates. This increased water loss must be balanced by increased water uptake from the soil. If water availability is limited, the plant will experience water stress, which can inhibit photosynthesis and growth. The plant hormone abscisic acid (ABA) plays a key role in coordinating the response to water stress. ABA triggers stomatal closure, which reduces transpiration but also limits carbon dioxide uptake for photosynthesis. Plants acclimate to drought through a number of complex molecular mechanism that can include reduced leaf area, altered root architecture and changes to cuticle properties.
5.2 Light and Nutrients
Light also affects nutrient uptake. High light intensity can increase the demand for nutrients, particularly nitrogen, which is a key component of chlorophyll. Plants grown under high light conditions may require higher levels of nitrogen fertilizer than plants grown under low light conditions. Conversely, nutrient deficiencies can limit photosynthesis, even under high light conditions. For example, nitrogen deficiency can reduce chlorophyll synthesis, limiting the plant’s ability to capture light energy.
5.3 Water and Nutrients
Water availability also affects nutrient uptake. Nutrients are transported to the roots in the soil water. Therefore, water stress can limit nutrient uptake, even if nutrients are present in the soil. Furthermore, water stress can inhibit root growth, reducing the surface area for nutrient absorption. Plants may form an association with mycorrhizal fungi to improve nutrient uptake under water-stressed conditions.
6. Emerging Research and Future Directions
Several emerging research areas hold promise for improving plant resource acquisition and performance. These include:
- Plant Hormones: Plant hormones play a crucial role in regulating plant growth and development, including resource acquisition. Research is ongoing to understand how hormones such as auxin, cytokinin, gibberellin, abscisic acid, and ethylene regulate processes such as root development, stomatal opening, and nutrient uptake. Manipulating hormone levels or signaling pathways could potentially enhance plant resource acquisition.
- Signaling Pathways: Plants use complex signaling pathways to sense and respond to changes in their environment. Understanding these signaling pathways could allow us to develop strategies for improving plant resilience to stress and optimizing resource acquisition. This could be particularly important in managed environments, where plants may be exposed to fluctuating conditions.
- The Microbiome: The plant microbiome, which consists of the microorganisms that live in and on plants, can play a significant role in plant health and resource acquisition. Research is ongoing to understand how the microbiome influences nutrient uptake, disease resistance, and stress tolerance. Manipulating the microbiome could potentially enhance plant performance.
- Genetic Engineering and CRISPR technology: The manipulation of plant genes and genomes may also provide a means of improving plant resource acquisition. For example, genes involved in root development or nutrient transport could be modified to enhance nutrient uptake. Genes involved in stomatal regulation could be modified to improve water use efficiency. The use of CRISPR-Cas9 technology offers the possibility of precise and targeted genome editing.
Future research should focus on integrating our understanding of plant physiology, molecular biology, and ecology to develop sustainable strategies for improving plant performance in both natural and managed ecosystems. This will require a multidisciplinary approach involving plant physiologists, molecular biologists, ecologists, and agricultural scientists.
7. Conclusion
The efficient acquisition of light, water, and nutrients is essential for plant growth and survival. These processes are interconnected and regulated by complex physiological mechanisms. Understanding these mechanisms is crucial for effective plant management, particularly in controlled environments where environmental parameters can be manipulated to enhance growth and productivity. Emerging research areas, such as the role of plant hormones, signaling pathways, and the microbiome in regulating resource acquisition, hold promise for improving plant performance in both natural and managed ecosystems. Further research is needed to integrate our understanding of plant physiology, molecular biology, and ecology to develop sustainable strategies for improving plant resource acquisition and resilience.
References
- Buchanan, B. B., Gruissem, W., & Jones, R. L. (2015). Biochemistry & molecular biology of plants (2nd ed.). John Wiley & Sons.
- Hopkins, W. G., & Hüner, N. P. A. (2009). Introduction to plant physiology (4th ed.). John Wiley & Sons.
- Jones, H. G. (2013). Plants and microclimate: A quantitative approach to environmental plant physiology (3rd ed.). Cambridge University Press.
- Marschner, H. (2012). Mineral nutrition of higher plants (3rd ed.). Academic Press.
- Taiz, L., Zeiger, E., Møller, I. M., & Murphy, A. (2018). Plant physiology and development (6th ed.). Sinauer Associates.
Be the first to comment