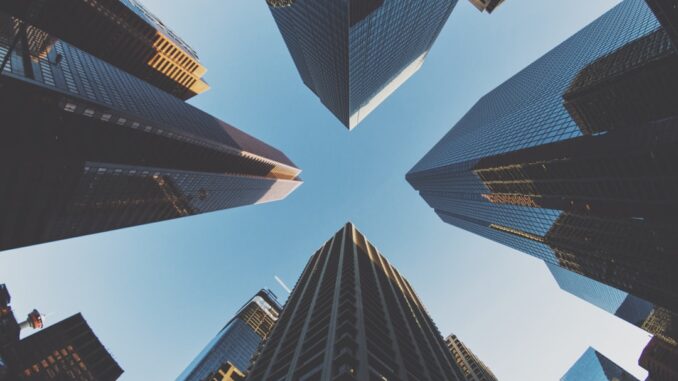
The Evolving Landscape of Sustainable Construction: Innovations, Challenges, and Future Directions
Abstract
The construction industry, a significant contributor to global environmental impact, is undergoing a transformative shift towards sustainable practices. This research report delves into the multifaceted landscape of sustainable construction, examining key innovations driving the sector, the persistent challenges hindering widespread adoption, and potential future directions. We analyze advancements in materials science, construction methodologies, and energy-efficient designs, alongside the economic, social, and regulatory barriers that impede progress. The report synthesizes existing literature and industry reports to provide a comprehensive overview of the state-of-the-art, identifying critical research gaps and outlining pathways for accelerating the transition towards a more sustainable and resilient built environment. A focus is put on the practical implications and limitations, not just the ideals. The aim is to provide experts with a nuanced view of the current state and potential future trajectories of sustainable construction.
1. Introduction: The Imperative for Sustainable Construction
The construction sector’s impact on the environment is undeniable. It consumes vast quantities of natural resources, contributes significantly to greenhouse gas emissions, and generates substantial waste (United Nations Environment Programme, 2020). Traditional construction practices, characterized by resource-intensive materials, inefficient energy usage, and linear waste management models, are unsustainable in the face of increasing environmental concerns and resource scarcity.
Sustainable construction, encompassing environmentally responsible and resource-efficient processes throughout a building’s lifecycle, is no longer merely a desirable aspiration but a fundamental imperative. It aims to minimize environmental impact, conserve resources, and create healthy and comfortable indoor environments, while also considering economic viability and social equity. This paradigm shift requires a holistic approach that integrates innovative technologies, design strategies, and policy frameworks.
This report examines the evolving landscape of sustainable construction, exploring the key drivers, technological advancements, and persistent challenges that shape the industry. By analyzing the current state of the art and identifying critical research gaps, this report aims to provide insights into the potential future directions of sustainable construction and contribute to accelerating its adoption worldwide.
2. Innovations in Sustainable Construction Materials
Materials play a critical role in the environmental footprint of a building. Sustainable construction necessitates the adoption of materials with lower embodied energy, reduced environmental impact, and enhanced durability. Several innovations are transforming the materials landscape:
2.1 Bio-Based Materials:
Bio-based materials, derived from renewable biological resources, offer a compelling alternative to conventional materials. Examples include:
- Timber: Responsibly sourced timber, particularly from sustainably managed forests, can sequester carbon dioxide and provide a renewable building material. Engineered wood products, such as cross-laminated timber (CLT) and glue-laminated timber (Glulam), offer enhanced strength and stability, expanding the applications of timber in large-scale construction (Ramage et al., 2017).
- Bamboo: A fast-growing and highly renewable resource, bamboo possesses impressive strength and versatility. It can be used in structural applications, cladding, and interior finishes. However, concerns remain regarding the durability and treatment of bamboo to prevent degradation (Sharma et al., 2015).
- Hempcrete: A mixture of hemp fibers, lime, and water, hempcrete offers excellent insulation properties, breathability, and carbon sequestration potential. While it is not a structural material, it can be used as infill in walls and roofs. The regulatory acceptance and cost-competitiveness of hempcrete remain challenges.
- Mycelium-based Materials: Utilizing the root structure of fungi, these materials offer a biodegradable alternative to traditional insulation and packaging materials. Research is ongoing to develop mycelium-based composites for structural applications, but significant scaling and performance hurdles remain.
2.2 Recycled and Reclaimed Materials:
Utilizing recycled and reclaimed materials reduces waste and conserves virgin resources. Examples include:
- Recycled Concrete Aggregate (RCA): Crushed concrete from demolition sites can be used as aggregate in new concrete mixes, reducing the demand for virgin aggregate. The quality and consistency of RCA are crucial considerations to ensure structural integrity (Xiao et al., 2012).
- Recycled Steel: Steel is highly recyclable, and recycled steel possesses comparable properties to virgin steel. Using recycled steel significantly reduces the energy required for production.
- Reclaimed Wood: Reclaimed wood from demolished buildings or salvaged timber offers a unique aesthetic and reduces the demand for newly harvested timber. However, it is important to ensure the structural integrity and absence of harmful chemicals in reclaimed wood.
- Recycled Plastics: Plastic waste can be recycled into various construction products, such as composite decking, insulation, and drainage systems. The durability and long-term performance of recycled plastic materials are important considerations.
2.3 Low-Carbon Cement and Concrete:
Cement production is a significant source of carbon dioxide emissions. Innovations in cement and concrete technology aim to reduce the carbon footprint of these essential building materials. These innovations include:
- Supplementary Cementitious Materials (SCMs): Replacing a portion of Portland cement with SCMs, such as fly ash, slag, and silica fume, reduces the overall carbon footprint of concrete. SCMs can also enhance the durability and performance of concrete (Lothenbach et al., 2011).
- Alternative Cements: Research is underway to develop alternative cements with lower carbon emissions, such as calcium sulfoaluminate (CSA) cement and alkali-activated materials (AAMs). These alternative cements offer promising potential, but further research is needed to optimize their performance and durability.
- Carbon Capture and Utilization (CCU): Technologies that capture carbon dioxide from industrial sources and utilize it in concrete production are emerging. CCU technologies can potentially sequester carbon dioxide and create value-added products, but their economic viability and scalability remain uncertain.
The choice of materials has a profound impact on the sustainability of a building project. By carefully selecting materials with lower embodied energy, recycled content, and renewable sources, designers and builders can significantly reduce the environmental footprint of the built environment. The use of these novel materials, however, often requires specialized knowledge and expertise, along with a thorough understanding of their long-term performance characteristics. Standards and codes must adapt to allow their use, and insurance companies must be confident to provide cover based on the use of less familiar materials.
3. Sustainable Construction Methodologies and Technologies
Beyond materials, the construction process itself plays a crucial role in sustainability. Innovative methodologies and technologies are transforming how buildings are designed, constructed, and operated:
3.1 Building Information Modeling (BIM):
BIM is a digital representation of a building’s physical and functional characteristics. It enables architects, engineers, and contractors to collaborate more effectively, optimize designs for energy efficiency, and identify potential conflicts before construction begins. BIM can also facilitate the tracking of materials, waste management, and lifecycle assessment.
3.2 Modular and Prefabricated Construction:
Modular and prefabricated construction involves manufacturing building components off-site in a controlled factory environment and then assembling them on-site. This approach offers several advantages, including reduced waste, improved quality control, faster construction times, and minimized disruption to surrounding areas. However, it requires careful planning and coordination to ensure seamless integration of the modules.
3.3 3D Printing:
3D printing, also known as additive manufacturing, is an emerging technology that can potentially revolutionize construction. It involves building structures layer by layer using materials such as concrete, polymers, or composites. 3D printing offers the potential for customized designs, reduced waste, and faster construction times. However, the technology is still in its early stages of development, and challenges remain regarding material selection, structural integrity, and regulatory acceptance.
3.4 Green Building Certifications:
Green building certifications, such as LEED (Leadership in Energy and Environmental Design) and BREEAM (Building Research Establishment Environmental Assessment Method), provide a framework for assessing and recognizing sustainable building practices. These certifications encourage the adoption of energy-efficient designs, water conservation measures, and healthy indoor environments. They also offer a benchmark for measuring and comparing the sustainability performance of different buildings. These certifications also push the innovation of products as companies strive to achieve higher ratings.
3.5 Digital Twins:
A digital twin is a virtual replica of a physical asset, such as a building or infrastructure system. It is updated in real-time with data from sensors, enabling performance monitoring, predictive maintenance, and optimized energy management. Digital twins can facilitate a more sustainable and resilient built environment by improving operational efficiency and extending the lifespan of buildings.
These methods and technologies offer potential to reduce waste and improve efficiency. But, the initial investment in these technologies can be a barrier to entry, particularly for smaller construction companies. Moreover, the integration of these technologies into existing workflows requires training and adaptation, which can be challenging. The reliance on digital tools also raises concerns about data security and privacy.
4. Challenges and Barriers to Sustainable Construction
Despite the growing awareness and technological advancements in sustainable construction, several challenges and barriers continue to impede its widespread adoption:
4.1 Cost Perceptions:
A common misconception is that sustainable construction is more expensive than conventional construction. While some sustainable materials and technologies may have a higher upfront cost, they can often result in lower lifecycle costs due to reduced energy consumption, water usage, and maintenance requirements. It is crucial to conduct a thorough lifecycle cost analysis to accurately assess the economic benefits of sustainable construction.
4.2 Lack of Awareness and Education:
Many stakeholders in the construction industry, including clients, developers, architects, and contractors, lack sufficient awareness and education about sustainable construction practices. This can lead to resistance to adopting new technologies and materials, as well as a failure to recognize the long-term benefits of sustainability. A better understanding of the long term ROI is critical.
4.3 Regulatory and Policy Barriers:
Inconsistent or outdated building codes and regulations can hinder the adoption of sustainable construction practices. Clear and consistent policies are needed to incentivize sustainable design, promote the use of sustainable materials, and enforce energy-efficient building standards. Permitting processes can also be slow and cumbersome, particularly for innovative construction methods.
4.4 Supply Chain Constraints:
The availability of sustainable materials and technologies can be limited in some regions. Supply chain constraints can increase costs and delay projects, making it difficult to implement sustainable construction practices. Stronger local supply chains are needed, as is confidence in the durability of products supplied.
4.5 Fragmentation and Collaboration:
The construction industry is often fragmented, with limited collaboration between different stakeholders. This can hinder the integration of sustainable design principles and the sharing of best practices. Improved communication and collaboration are essential to promote a more holistic and sustainable approach to construction.
Addressing these challenges requires a multi-faceted approach involving education, policy incentives, industry collaboration, and technological innovation. Furthermore, it is essential to recognize that sustainability is not a one-size-fits-all solution, and that different regions and building types may require tailored approaches. The specific challenges and priorities can vary significantly depending on local climate, resource availability, and cultural context. Addressing these unique conditions is essential to ensuring the long-term success of sustainable construction initiatives.
5. Future Directions and Research Needs
The future of sustainable construction holds immense potential. Several key trends and research areas are likely to shape the industry in the coming years:
5.1 Circular Economy Principles:
The construction industry is increasingly adopting circular economy principles, aiming to minimize waste, maximize resource utilization, and extend the lifespan of buildings. This involves designing buildings for disassembly and reuse, promoting the use of recycled and reclaimed materials, and implementing closed-loop waste management systems. Building designs must evolve, taking into account the end of life of the building.
5.2 Smart Buildings and IoT:
The integration of smart technologies and the Internet of Things (IoT) is transforming how buildings are operated and managed. Smart buildings can automatically adjust lighting, heating, and cooling based on occupancy and environmental conditions, optimizing energy consumption and improving occupant comfort. IoT sensors can also monitor building performance, detect potential problems, and facilitate predictive maintenance.
5.3 Data-Driven Sustainability:
The availability of large datasets and advanced data analytics techniques is enabling a more data-driven approach to sustainability. Data can be used to optimize building designs for energy efficiency, track material usage, and monitor the environmental impact of construction projects. Machine learning algorithms can also be used to predict building performance and identify opportunities for improvement.
5.4 Nature-Based Solutions:
Nature-based solutions, such as green roofs, green walls, and urban forests, are gaining increasing attention as a way to enhance the sustainability and resilience of buildings and cities. These solutions can reduce stormwater runoff, mitigate the urban heat island effect, improve air quality, and enhance biodiversity.
5.5 Further Research and Development:
Further research and development are needed in several areas to accelerate the transition to sustainable construction, including:
- Developing new and improved sustainable materials with enhanced performance and durability.
- Optimizing building designs for energy efficiency, water conservation, and passive solar heating and cooling.
- Developing innovative construction methodologies and technologies that reduce waste and improve productivity.
- Improving the lifecycle assessment of buildings and construction materials.
- Developing standardized metrics and frameworks for measuring and reporting sustainability performance.
- Investigating the social and economic impacts of sustainable construction practices.
In addition to these specific research areas, there is a need for more interdisciplinary research that integrates expertise from engineering, architecture, environmental science, economics, and social sciences. This collaborative approach is essential to address the complex challenges of sustainable construction and develop holistic solutions that benefit society as a whole. The current reliance on pilot projects and case studies needs to be complemented by more rigorous, large-scale studies that can provide statistically significant evidence of the benefits of sustainable construction practices. This will help to build confidence in the effectiveness of these practices and encourage their widespread adoption.
6. Conclusion
Sustainable construction is rapidly evolving, driven by growing environmental concerns, technological advancements, and policy initiatives. While significant progress has been made in developing sustainable materials, methodologies, and technologies, numerous challenges remain. Addressing these challenges requires a concerted effort from all stakeholders, including governments, industry, academia, and the public. By embracing innovation, fostering collaboration, and investing in research and education, the construction industry can play a crucial role in creating a more sustainable and resilient built environment for future generations.
The transition to sustainable construction is not merely a technical challenge; it is a societal transformation that requires a shift in mindset and values. It requires a recognition that buildings are not simply commodities, but integral components of a complex and interconnected ecosystem. The industry must move beyond a focus on short-term profits and embrace a longer-term perspective that considers the environmental, social, and economic impacts of its activities. This requires a fundamental rethinking of the way buildings are designed, constructed, operated, and ultimately, deconstructed.
References
- Lothenbach, B., Scrivener, K., & Hooton, R. D. (2011). Supplementary cementitious materials. Cement and Concrete Research, 41(12), 1244-1256.
- Ramage, M. H., Burridge, H., Busse-Wicher, M., Fereday, K. J., Reynolds, T., Shah, D. U., … & Allin, S. (2017). The wood from the trees: The use of timber in construction. Renewable and Sustainable Energy Reviews, 68, 344-359.
- Sharma, B., Gatóo, A., Bock, M., & Ramage, M. (2015). Sustainable bamboo reinforced concrete for structural applications. Materials & Design, 68, 86-101.
- United Nations Environment Programme. (2020). 2020 Global Status Report for Buildings and Construction: Towards a Zero-emission, Efficient and Resilient Buildings and Construction Sector. Nairobi.
- Xiao, J., Li, W., Poon, C. S., Hui, D., & Falkner, F. (2012). Recent studies on mechanical properties of recycled aggregate concrete. Construction and Building Materials, 29, 691-700.
This report highlights the importance of sustainable materials. What advancements do you see in integrating local, readily available resources like earth or agricultural byproducts to minimize transportation impacts and support regional economies?
Interesting stuff! Dare I suggest focusing less on *mitigating* impact and more on construction that actively benefits ecosystems? Imagine buildings that purify water or enhance biodiversity. Now *that’s* a landscape I’d like to see evolve.
The report mentions integrating digital twins for performance monitoring. Could this technology be further leveraged during the design phase to simulate environmental impacts and optimize material selection before construction even begins?
The emphasis on lifecycle assessment is key. How can we better integrate predictive models, incorporating climate change scenarios, to assess the long-term durability and adaptability of sustainable materials in diverse regional contexts?
Fascinating report! Given the emphasis on lifecycle, should we also be exploring incentivizing deconstruction and material reuse at the *end* of a building’s life, perhaps through tax breaks or credits? Imagine a future where buildings are designed not just for construction, but for elegant disassembly.
This is insightful! How can the industry standardize lifecycle assessments to ensure consistent and comparable data across different building projects, enabling more informed decision-making and benchmarking?
So, we’re thinking about buildings that disassemble elegantly? I’m picturing a giant LEGO set. Maybe we should focus on universal connection points so reclaimed components can slot seamlessly into new designs. Think of the possibilities!
Given the focus on the construction industry’s environmental impact, I wonder how effectively current sustainability initiatives address the embedded carbon footprint of existing building stock versus new construction? Can retrofitting strategies truly offset the environmental debt of older, less efficient structures?
Considering the report’s call for standardized metrics, how might we leverage blockchain technology to ensure transparency and traceability in the sourcing and certification of sustainable construction materials, enhancing trust and accountability across the supply chain?
So, are we thinking enough about the skills gap this tech revolution creates? Will we need an army of AI whisperers to keep these smart buildings from staging a revolt against inefficient humans?
Given the emphasis on lifecycle cost analysis versus upfront costs, how can governments and industry collaborate to create financial models that better reflect the long-term economic benefits of sustainable construction, potentially through subsidies or tax incentives?