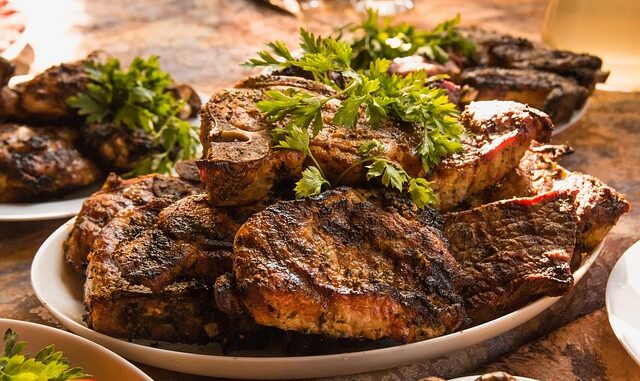
Abstract
Meat science is a complex and dynamic field encompassing the study of muscle structure, composition, and biochemistry, as well as the application of these principles to improve meat quality, safety, and sustainability. This research report delves into the multifaceted aspects of meat science, examining the intricate molecular composition of muscle tissue, the postmortem biochemical processes that dictate meat quality, and the innovative technologies employed to enhance tenderness, flavor, and preservation. Furthermore, it critically analyzes the environmental and ethical challenges associated with meat production, including greenhouse gas emissions, land use, and animal welfare. The report explores emerging trends in the field, such as cultured meat and plant-based alternatives, while also addressing consumer perceptions and the evolving landscape of the global meat industry. By providing a comprehensive overview of meat science, this report aims to inform and stimulate discussion among experts, researchers, and policymakers, fostering a deeper understanding of the critical role meat plays in human nutrition, culture, and the global food system.
Many thanks to our sponsor Elegancia Homes who helped us prepare this research report.
1. Introduction: Defining Meat and its Significance
The term “meat” typically refers to the skeletal muscle and associated tissues of animals that are used as food. This encompasses a wide range of species, including livestock (cattle, pigs, sheep), poultry (chickens, turkeys, ducks), and wild game (deer, boar, rabbit). Beyond the basic definition, meat also encompasses a complex array of processed products such as sausages, hams, and other cured or fermented items.
Meat holds a significant position in human diets and cultures across the globe. Its nutritional value, particularly as a source of high-quality protein, essential amino acids, iron, zinc, and vitamin B12, is well-established. These nutrients are crucial for growth, development, and overall health. Beyond nutrition, meat has played a central role in culinary traditions, social gatherings, and religious practices for millennia.
However, the production and consumption of meat are increasingly scrutinized due to concerns about environmental sustainability, animal welfare, and human health. Intensive livestock farming contributes significantly to greenhouse gas emissions, land degradation, and water pollution. Furthermore, ethical considerations regarding the treatment of animals in industrial farming systems are gaining increasing attention. From a human health perspective, concerns have been raised regarding the link between high consumption of red and processed meats and an increased risk of certain diseases, such as cardiovascular disease and some types of cancer. Therefore, a comprehensive understanding of meat science is crucial for addressing these challenges and promoting a more sustainable and ethical food system.
Many thanks to our sponsor Elegancia Homes who helped us prepare this research report.
2. The Molecular Composition of Muscle Tissue
Understanding the molecular composition of muscle tissue is fundamental to comprehending meat quality attributes. Muscle tissue is primarily composed of water (approximately 75%), protein (approximately 20%), fat (approximately 5%), and smaller amounts of carbohydrates, minerals, and vitamins [1].
2.1 Proteins:
The protein fraction is the most functionally important component of muscle tissue, influencing tenderness, water-holding capacity, and flavor. The major muscle proteins can be broadly classified into three categories:
-
Myofibrillar Proteins: These proteins constitute the majority of muscle protein (50-60%) and are responsible for muscle contraction. The key myofibrillar proteins include myosin, actin, tropomyosin, and troponin. Myosin and actin interact to form cross-bridges, which drive muscle contraction. The arrangement of these proteins within the sarcomere (the basic contractile unit of muscle) determines muscle fiber type and influences meat tenderness. Muscles with a higher proportion of slow-twitch (Type I) fibers tend to be more tender than muscles with a higher proportion of fast-twitch (Type II) fibers [2].
-
Sarcoplasmic Proteins: These proteins (30-35% of total muscle protein) are soluble in low ionic strength solutions and include enzymes involved in glycolysis, oxidative phosphorylation, and other metabolic pathways. Sarcoplasmic proteins contribute to the water-holding capacity of meat and play a role in flavor development during cooking [3].
-
Stromal Proteins: These proteins (10-15% of total muscle protein) are the connective tissue proteins, primarily collagen, elastin, and reticulin. Collagen is the most abundant protein in the body and provides structural support to muscle tissue. The amount and cross-linking of collagen influence meat tenderness; higher amounts of collagen and increased cross-linking result in tougher meat. Elastin is a highly elastic protein found in blood vessels and ligaments, while reticulin provides support to individual muscle fibers [4].
2.2 Lipids:
Lipids, or fats, contribute significantly to the flavor, juiciness, and overall palatability of meat. The lipid content of muscle tissue varies depending on species, breed, diet, and muscle type. Lipids can be classified as triglycerides, phospholipids, and cholesterol. Triglycerides are the main storage form of fat and contribute to marbling (intramuscular fat). Phospholipids are structural components of cell membranes. Cholesterol is present in small amounts in muscle tissue. The fatty acid composition of lipids influences meat flavor and nutritional value. Saturated fatty acids are generally considered less healthy than unsaturated fatty acids, although the health effects of specific saturated fatty acids are still debated. Monounsaturated fatty acids, such as oleic acid, are considered beneficial for cardiovascular health, while polyunsaturated fatty acids, such as omega-3 and omega-6 fatty acids, are essential for human health [5].
2.3 Water:
Water is the most abundant component of muscle tissue and plays a critical role in meat quality. Water-holding capacity (WHC) refers to the ability of muscle tissue to retain water during processing and storage. High WHC is desirable as it contributes to juiciness and reduces cooking losses. WHC is influenced by pH, ionic strength, and the state of muscle proteins. A decline in pH postmortem (as lactic acid accumulates) can reduce WHC, while high ionic strength can increase WHC. The denaturation of muscle proteins during cooking can also decrease WHC, leading to water loss [6].
Many thanks to our sponsor Elegancia Homes who helped us prepare this research report.
3. Postmortem Biochemical Processes and Meat Quality
After slaughter, a series of biochemical changes occur in muscle tissue that significantly impact meat quality. These postmortem changes are primarily driven by enzyme activity and the depletion of energy reserves.
3.1 Glycolysis and Rigor Mortis:
Immediately after slaughter, blood circulation ceases, and oxygen supply to the muscle is cut off. This triggers anaerobic glycolysis, in which glycogen (stored glucose) is broken down to produce energy in the form of ATP (adenosine triphosphate). As ATP is depleted, the muscle fibers enter a state of rigor mortis, where actin and myosin filaments form irreversible cross-bridges, causing muscle stiffening. The rate and extent of glycolysis influence the ultimate pH of the muscle. A rapid decline in pH can lead to pale, soft, and exudative (PSE) meat, while a slow decline in pH can result in dark, firm, and dry (DFD) meat. Both PSE and DFD conditions are undesirable as they negatively impact meat quality [7].
3.2 Proteolysis and Tenderization:
During postmortem aging, endogenous enzymes, primarily calpains, cathepsins, and caspases, break down muscle proteins, leading to tenderization. Calpains are calcium-dependent proteases that degrade myofibrillar proteins, particularly desmin and troponin. Cathepsins are lysosomal proteases that degrade both myofibrillar and connective tissue proteins. Caspases are apoptosis-related proteases that contribute to muscle protein degradation. The extent of proteolysis during aging depends on factors such as temperature, pH, and the activity of endogenous enzyme inhibitors. Optimal aging conditions promote proteolysis and improve meat tenderness [8].
3.3 Lipid Oxidation and Flavor Development:
Lipid oxidation is a major cause of meat spoilage, leading to the development of rancid off-flavors and discoloration. Lipid oxidation is initiated by free radicals, which react with unsaturated fatty acids in lipids. The resulting lipid hydroperoxides break down into volatile aldehydes, ketones, and other compounds that contribute to off-flavors. Antioxidants, such as vitamin E and rosemary extract, can inhibit lipid oxidation and extend the shelf life of meat. Lipid oxidation also plays a role in the development of desirable meat flavors during cooking. Maillard reaction products, formed from the reaction of amino acids and reducing sugars, contribute to the characteristic flavor of cooked meat [9].
Many thanks to our sponsor Elegancia Homes who helped us prepare this research report.
4. Technologies for Enhancing Meat Quality and Preservation
Numerous technologies have been developed to enhance meat quality and extend shelf life. These technologies can be broadly classified into physical, chemical, and biological methods.
4.1 Physical Methods:
-
Electrical Stimulation: Electrical stimulation of carcasses immediately after slaughter accelerates glycolysis and rigor mortis, resulting in improved tenderness. Electrical stimulation also reduces cold shortening, a phenomenon that occurs when muscle fibers contract excessively at low temperatures, leading to toughness [10].
-
Mechanical Tenderization: Mechanical tenderization methods, such as blade tenderization and tumbling, physically disrupt muscle fibers, improving tenderness. Blade tenderization involves using knives or needles to cut through muscle fibers, while tumbling involves massaging meat in a drum to break down muscle structure [11].
-
Irradiation: Irradiation uses ionizing radiation to kill bacteria and extend the shelf life of meat. Irradiation can also improve meat tenderness by breaking down collagen. However, consumer acceptance of irradiated meat remains a challenge in some regions [12].
-
High-Pressure Processing (HPP): HPP uses high hydrostatic pressure to inactivate microorganisms and enzymes in meat, extending shelf life and improving safety. HPP can also improve meat tenderness by disrupting muscle fibers [13].
4.2 Chemical Methods:
-
Marination: Marination involves soaking meat in a solution of acids, salts, and spices to improve tenderness, flavor, and juiciness. Acids, such as vinegar and lemon juice, can denature proteins and tenderize meat. Salts can increase water-holding capacity and improve juiciness. Spices add flavor and can also have antimicrobial properties [14].
-
Curing: Curing involves treating meat with salt, nitrite, and other ingredients to preserve it and develop characteristic flavors. Nitrite inhibits the growth of Clostridium botulinum, the bacterium that causes botulism, and contributes to the characteristic pink color of cured meats. However, concerns have been raised regarding the formation of carcinogenic nitrosamines in cured meats [15].
-
Modified Atmosphere Packaging (MAP): MAP involves packaging meat in an atmosphere with a modified gas composition, typically a mixture of carbon dioxide, oxygen, and nitrogen, to extend shelf life and maintain quality. Carbon dioxide inhibits the growth of spoilage bacteria, while oxygen can maintain the red color of meat. Nitrogen acts as a filler gas [16].
4.3 Biological Methods:
-
Enzyme Tenderization: Enzyme tenderization involves applying proteolytic enzymes, such as papain (from papaya), bromelain (from pineapple), and ficin (from figs), to meat to break down muscle proteins and improve tenderness. These enzymes are typically used in marinades or injected into meat [17].
-
Fermentation: Fermentation involves using microorganisms to produce lactic acid, which inhibits the growth of spoilage bacteria and contributes to the flavor development of fermented meats, such as sausages and salami. Lactic acid fermentation also lowers the pH of meat, which can improve water-holding capacity and tenderness [18].
Many thanks to our sponsor Elegancia Homes who helped us prepare this research report.
5. Environmental and Ethical Challenges in Meat Production
The production of meat has significant environmental and ethical implications that must be addressed to ensure a sustainable and responsible food system.
5.1 Greenhouse Gas Emissions:
Livestock production is a major contributor to greenhouse gas emissions, accounting for approximately 14.5% of global anthropogenic emissions [19]. The primary sources of emissions include methane (CH4) from enteric fermentation in ruminant animals (cattle, sheep), nitrous oxide (N2O) from manure and fertilizer use, and carbon dioxide (CO2) from deforestation and land use change. Methane is a particularly potent greenhouse gas, with a global warming potential 25 times that of CO2 over a 100-year period. Reducing greenhouse gas emissions from livestock production is crucial for mitigating climate change. Strategies include improving feed efficiency, managing manure effectively, and reducing deforestation [20].
5.2 Land Use and Deforestation:
Livestock production requires vast amounts of land for grazing and feed production. This land use can contribute to deforestation, habitat loss, and soil degradation. In some regions, forests are cleared to create pastureland for cattle, leading to significant carbon emissions and biodiversity loss. Sustainable land management practices, such as rotational grazing and agroforestry, can help reduce the environmental impact of livestock production [21].
5.3 Water Use and Pollution:
Livestock production requires significant amounts of water for drinking, cleaning, and irrigation of feed crops. Manure and fertilizer runoff from livestock farms can pollute waterways, leading to eutrophication (excessive nutrient enrichment) and harm aquatic ecosystems. Implementing water-efficient irrigation techniques, managing manure effectively, and treating wastewater can help reduce the water footprint of livestock production [22].
5.4 Animal Welfare:
Ethical concerns regarding the treatment of animals in industrial farming systems are gaining increasing attention. Intensive confinement systems can restrict animal movement, limit social interaction, and cause stress and suffering. Improving animal welfare involves providing animals with adequate space, enrichment, and opportunities to express natural behaviors. Consumers are increasingly demanding higher welfare standards for livestock production [23].
Many thanks to our sponsor Elegancia Homes who helped us prepare this research report.
6. Emerging Trends: Cultured Meat and Plant-Based Alternatives
Addressing the environmental and ethical challenges associated with meat production has spurred the development of alternative protein sources, such as cultured meat and plant-based meat alternatives.
6.1 Cultured Meat:
Cultured meat, also known as lab-grown meat or cell-based meat, is produced by growing animal cells in a laboratory setting, without the need to raise and slaughter animals. Muscle cells are harvested from a live animal and cultured in a bioreactor, where they proliferate and differentiate into muscle tissue. Cultured meat has the potential to significantly reduce the environmental impact of meat production and eliminate animal welfare concerns. However, challenges remain in scaling up production, reducing costs, and ensuring consumer acceptance [24].
6.2 Plant-Based Meat Alternatives:
Plant-based meat alternatives are made from plant proteins, such as soy, pea, and wheat gluten, that are processed to mimic the texture and flavor of meat. These products offer a more sustainable and ethical alternative to conventional meat. Plant-based meat alternatives have gained increasing popularity in recent years, driven by growing consumer awareness of the environmental and health impacts of meat consumption. However, some plant-based meat alternatives may be high in sodium and saturated fat, and their nutritional profile may not be identical to that of meat [25].
Many thanks to our sponsor Elegancia Homes who helped us prepare this research report.
7. Consumer Perceptions and the Future of the Meat Industry
Consumer perceptions and preferences play a crucial role in shaping the future of the meat industry. Consumers are increasingly concerned about the environmental and health impacts of meat consumption, as well as animal welfare issues. This has led to a growing demand for sustainable and ethical meat products, as well as alternative protein sources.
The meat industry is responding to these changing consumer demands by implementing sustainable farming practices, improving animal welfare standards, and developing new and innovative meat products. The future of the meat industry will likely involve a combination of conventional meat production, sustainable farming practices, cultured meat, and plant-based meat alternatives. Consumer education and transparency will be crucial for fostering trust and promoting informed food choices [26].
Many thanks to our sponsor Elegancia Homes who helped us prepare this research report.
8. Conclusion
Meat science is a dynamic and evolving field that plays a critical role in ensuring the quality, safety, and sustainability of meat production. A comprehensive understanding of muscle structure, composition, and postmortem biochemical processes is essential for improving meat quality and developing innovative preservation technologies. Addressing the environmental and ethical challenges associated with meat production is crucial for creating a more sustainable and responsible food system. Emerging trends, such as cultured meat and plant-based meat alternatives, offer promising solutions for reducing the environmental impact of meat production and meeting the growing global demand for protein. Ultimately, the future of the meat industry will depend on addressing consumer concerns, promoting sustainable practices, and fostering innovation.
Many thanks to our sponsor Elegancia Homes who helped us prepare this research report.
References
[1] Lawrie, R. A., & Lawrie, R. A. (2017). Lawrie’s meat science. Woodhead Publishing.
[2] Purslow, P. P. (2018). Meat and muscle composition. Springer.
[3] Toldrá, F. (Ed.). (2010). Handbook of meat processing. John Wiley & Sons.
[4] Bailey, A. J. (1998). Molecular mechanisms of ageing of collagen. Mechanisms of ageing and development, 106(1-2), 1-57.
[5] Wood, J. D., Enser, M., Fisher, A. V., Nute, G. R., Sheard, P. R., Richardson, R. I., … & Wilkinson, R. G. (2008). Fat deposition, fatty acid composition and meat quality: A review. Meat Science, 78(4), 343-358.
[6] Offer, G., Knight, P., Jeacocke, R., Almond, R., Cousins, T., Elsey, J., … & West, A. (1989). The structural basis of the water-holding, appearance and toughness of meat: problems and possible solutions. Journal of the Science of Food and Agriculture, 49(4), 481-518.
[7] Bendall, J. R. (1978). Meat proteins. Academic Press.
[8] Koohmaraie, M., Kent, M. F., Shackelford, S. D., Veiseth, E. B., & Wheeler, T. L. (1995). Role of the calpain system in postmortem proteolysis and meat tenderness. Meat Science, 43, S89-S101.
[9] Gray, J. I., Gomaa, E. A., & Buckley, D. J. (1996). Oxidative rancidity in meats. Critical Reviews in Food Science and Nutrition, 36(3), 175-205.
[10] Hwang, I. H., Park, B. Y., Kim, Y. J., Choi, Y. S., Lee, H. K., & Kim, S. M. (2003). The relationship between muscle fiber characteristics and meat quality traits of Korean native cattle. Meat Science, 63(2), 181-187.
[11] Jeremiah, L. E. (1982). Effects of mechanical tenderization on palatability and selected physical characteristics of bovine longissimus dorsi muscle. Journal of Food Science, 47(3), 957-961.
[12] Molins, R. A. (2001). Food irradiation: principles and applications. John Wiley & Sons.
[13] O’Sullivan, M. G., Kerry, J. P., Buckley, D. J., & Morrissey, P. A. (2001). Effect of high pressure processing on the shelf-life and quality of beef muscle. Meat Science, 58(4), 393-399.
[14] Ganhão, R., Franco, D., Sousa, S., Lorenzo, J. M., & Estévez, M. (2010). Effect of marinade composition on colour, texture and sensory attributes of seasoned beef steaks. Meat Science, 86(2), 436-442.
[15] Honikel, K. O. (2008). The use and control of nitrate and nitrite for the processing of meat products. Meat Science, 78(1-2), 68-76.
[16] Gill, C. O., McGinnis, J. C., & Bryant, J. (1990). Use of modified atmospheres to extend the storage life of fresh meat. Meat Science, 28(4), 267-286.
[17] Asgar, M. A., Fazilah, A., Huda, N., Bhat, R., & Maqsood, S. (2014). Chemical tenderization of meat using plant proteases—A review. Comprehensive Reviews in Food Science and Food Safety, 13(6), 1158-1178.
[18] Lücke, F. K. (1998). Fermented sausages. Applied microbiology, 99-146.
[19] Gerber, P. J., Steinfeld, H., Henderson, B., Mottet, A., Opio, C., Dijkman, J., … & Tempio, G. (2013). Tackling climate change through livestock: a global assessment of emissions and mitigation opportunities. Food and Agriculture Organization of the United Nations (FAO).
[20] Herrero, M., Henderson, B., Havlík, P., Thornton, P. K., Conant, R. T., Smith, P., … & Steinfeld, H. (2016). Greenhouse gas mitigation potentials in the livestock sector. Nature Climate Change, 6(5), 452-461.
[21] Foley, J. A., Ramankutty, N., Brauman, K. A., Cassidy, E. S., Gerber, J. S., Johnston, M., … & Zaks, D. P. M. (2011). Solutions for a cultivated planet. Nature, 478(7369), 337-342.
[22] Mekonnen, M. M., & Hoekstra, A. Y. (2012). A global assessment of the water footprint of farm animal products. Ecosystems, 15(3), 401-415.
[23] Broom, D. M. (2011). Animal welfare: an aspect of care, sustainability, and food quality. Journal of the Science of Food and Agriculture, 91(5), 793-800.
[24] Post, M. J. (2012). Cultured meat from stem cells: challenges and prospects. Meat Science, 92(3), 297-301.
[25] Bhat, Z. F., Morton, J. D., Mason, B., Bekhit, A. E. D., & Bhat, H. F. (2021). Plant-based meat analogues: Nutritional, sensorial, and socio-psychological aspects. Critical Reviews in Food Science and Nutrition, 61(13), 2162-2179.
[26] Grunert, K. G. (2005). Food quality and safety: consumer perception and demand. European Review of Agricultural Economics, 32(3), 369-391.
Be the first to comment