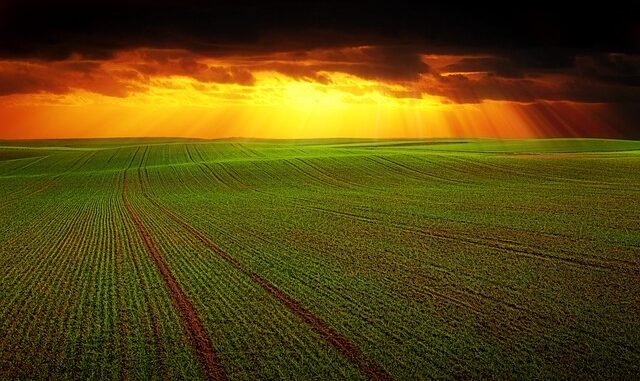
Abstract
Plant secondary metabolites (PSMs) are a diverse group of organic compounds synthesized by plants that are not directly involved in their growth, development, or reproduction, but play crucial roles in plant defense, environmental adaptation, and interaction with other organisms. This research report delves into the intricate relationship between PSMs, crop health, and human nutrition, examining the influence of agricultural practices, environmental factors, and genetic manipulation on PSM profiles in food crops. We will explore the potential of manipulating PSM production to enhance crop resistance to biotic and abiotic stresses, improve post-harvest quality, and bolster the nutritional value of food. Furthermore, we will discuss the challenges associated with characterizing and quantifying PSMs, understanding their complex interactions, and translating this knowledge into practical applications for sustainable agriculture and improved human health. Finally, we will consider the regulatory landscape surrounding PSMs in food and the ethical considerations related to their manipulation.
Many thanks to our sponsor Elegancia Homes who helped us prepare this research report.
1. Introduction
The increasing global population and the growing prevalence of chronic diseases necessitate a multifaceted approach to improve food security and human health. While traditional agricultural practices have focused primarily on yield maximization, the importance of nutritional quality and the inherent health benefits of food are gaining increasing recognition. Plant secondary metabolites (PSMs) are a pivotal link between plant health, agricultural sustainability, and human well-being. These compounds, produced through intricate biochemical pathways, provide plants with a range of adaptive advantages, including defense against pathogens, herbivores, and environmental stressors such as UV radiation and drought. Concurrently, PSMs contribute significantly to the organoleptic properties of food (taste, color, aroma) and impart a wide array of health-promoting effects, including antioxidant, anti-inflammatory, anticancer, and cardioprotective properties.
The focus of this report is to explore the current state of knowledge regarding PSMs, their manipulation in crops, and the translation of this knowledge into practical applications. We will examine the factors that influence PSM production, including genetic background, environmental conditions, and agricultural practices. Furthermore, we will explore the potential of genetic engineering, metabolic engineering, and other biotechnological tools to enhance PSM content in food crops. We will also address the challenges associated with PSM research, including the complexity of metabolic pathways, the lack of comprehensive databases, and the difficulties in assessing the bioavailability and bioactivity of PSMs in humans. Finally, we will discuss the regulatory and ethical considerations surrounding the use of PSMs in food and medicine.
Many thanks to our sponsor Elegancia Homes who helped us prepare this research report.
2. Biosynthesis and Classification of Plant Secondary Metabolites
PSMs are synthesized through a variety of biochemical pathways, typically branching off from primary metabolic pathways. The major classes of PSMs include:
-
Terpenoids: Derived from isopentenyl pyrophosphate (IPP) and dimethylallyl pyrophosphate (DMAPP), terpenoids are a diverse group of compounds including monoterpenes (e.g., menthol, limonene), sesquiterpenes (e.g., artemisinin), diterpenes (e.g., taxol), triterpenes (e.g., sterols, saponins), and tetraterpenes (e.g., carotenoids). Terpenoids play roles in plant defense, allelopathy, and signaling, and possess a variety of medicinal properties, including anticancer and anti-inflammatory activities.
-
Phenolics: Synthesized via the shikimate, phenylpropanoid, and acetate-malonate pathways, phenolics are characterized by the presence of one or more aromatic rings bearing hydroxyl substituents. This group includes flavonoids (e.g., anthocyanins, quercetin), phenolic acids (e.g., caffeic acid, ferulic acid), lignans, tannins, and coumarins. Phenolics are involved in plant defense against UV radiation and pathogens, and exhibit antioxidant, anti-inflammatory, and cardioprotective properties in humans.
-
Nitrogen-containing compounds: This class includes alkaloids (e.g., morphine, caffeine, nicotine), cyanogenic glycosides (e.g., amygdalin), glucosinolates (e.g., sinigrin), and non-protein amino acids. Alkaloids are often potent toxins that deter herbivores and possess a wide range of pharmacological activities. Cyanogenic glycosides and glucosinolates release cyanide and isothiocyanates, respectively, upon hydrolysis, providing a defense mechanism against pests and pathogens.
The synthesis of PSMs is tightly regulated by a complex interplay of genetic and environmental factors. The expression of genes encoding enzymes involved in PSM biosynthesis is influenced by developmental stage, tissue type, and exposure to biotic and abiotic stresses. Understanding the regulatory mechanisms governing PSM biosynthesis is crucial for manipulating PSM production in crops.
Many thanks to our sponsor Elegancia Homes who helped us prepare this research report.
3. Factors Influencing PSM Production in Crops
Several factors influence the biosynthesis and accumulation of PSMs in crops:
-
Genetic Factors: The genetic makeup of a plant species or variety determines its inherent capacity to synthesize specific PSMs. Variations in gene sequence, gene expression, and enzyme activity can lead to significant differences in PSM profiles among different cultivars. Plant breeding and genetic engineering can be used to select for or introduce genes that enhance PSM production.
-
Environmental Factors: Environmental conditions, such as light intensity, temperature, water availability, and nutrient availability, can significantly influence PSM biosynthesis. For example, exposure to UV radiation can stimulate the production of flavonoids, which act as UV protectants. Similarly, drought stress can induce the accumulation of proline and other osmolytes, as well as defensive compounds such as glucosinolates. Temperature also plays a critical role as low temperatures trigger accumulation of anthocyanins which act as a protective mechanism.
-
Agricultural Practices: Agricultural practices, such as fertilization, irrigation, pest control, and harvesting methods, can also affect PSM production. For example, nitrogen fertilization can decrease the synthesis of certain PSMs, as plants prioritize primary metabolism over secondary metabolism under conditions of high nutrient availability. Furthermore, the application of pesticides can alter PSM profiles by inducing defense responses in plants.
-
Biotic Interactions: Interactions with other organisms, such as pathogens, herbivores, and beneficial microbes, can trigger the production of PSMs. Plants can recognize specific elicitors produced by pathogens and herbivores, leading to the activation of defense pathways and the synthesis of antimicrobial or anti-herbivore compounds. Beneficial microbes, such as mycorrhizal fungi and plant growth-promoting rhizobacteria (PGPR), can also induce the production of PSMs, enhancing plant resistance to stress and promoting plant growth. An example is the production of phytoalexins.
Understanding the interplay of these factors is critical for optimizing agricultural practices to enhance PSM content in crops and improve their nutritional value and resilience. This is not a simple undertaking and the complexity of these interactions means that a holistic approach to optimising PSM production in crops is required.
Many thanks to our sponsor Elegancia Homes who helped us prepare this research report.
4. Strategies for Enhancing PSM Content in Crops
Several strategies can be employed to enhance PSM content in crops:
-
Plant Breeding: Traditional plant breeding techniques can be used to select for cultivars with naturally high levels of desirable PSMs. Marker-assisted selection (MAS) can accelerate the breeding process by allowing breeders to identify plants carrying specific genes associated with PSM production. For instance, breeders might focus on selecting varieties naturally resistant to pests, which would reduce the need for pesticide application and potentially enhance the natural accumulation of defensive PSMs.
-
Genetic Engineering: Genetic engineering offers the potential to introduce or enhance the expression of genes involved in PSM biosynthesis. This can be achieved by transforming plants with genes encoding rate-limiting enzymes in the biosynthetic pathway, or by manipulating regulatory genes that control the expression of multiple genes in the pathway. For instance, genes encoding transcription factors that upregulate flavonoid biosynthesis could be introduced into plants to increase their antioxidant capacity.
-
Metabolic Engineering: Metabolic engineering involves the rational design and optimization of metabolic pathways to enhance the production of specific PSMs. This can be achieved by manipulating enzyme activity, substrate availability, or pathway regulation. For example, precursor feeding strategies can be used to provide plants with increased amounts of substrates required for PSM biosynthesis. This approach requires a thorough understanding of the metabolic network and the regulatory mechanisms controlling flux through the pathway. However, potential bottlenecks such as precursor availability must be addressed.
-
Elicitation: Elicitation involves the application of biotic or abiotic elicitors to plants to stimulate the production of PSMs. Biotic elicitors can include microbial cell wall fragments, oligosaccharides, or peptides. Abiotic elicitors can include UV radiation, ozone, or heavy metals. Elicitation can be applied exogenously, by spraying plants with elicitor solutions, or endogenously, by inducing systemic acquired resistance (SAR) or induced systemic resistance (ISR). However, elicitation can also have unintended consequences, such as reduced growth or altered plant metabolism.
-
Optimized Agricultural Practices: Modifying agricultural practices, such as fertilization, irrigation, and pest control, can also enhance PSM content in crops. For example, reducing nitrogen fertilization can promote the synthesis of certain PSMs, while applying organic fertilizers can enhance soil microbial activity and promote plant growth. Careful management of water stress can also enhance the production of certain PSMs, but it is important to avoid excessive stress that can reduce yield. Integrated pest management (IPM) strategies can reduce the need for pesticide application and promote the natural accumulation of defensive PSMs. The ideal approach to optimizing agricultural practices will be highly crop specific.
These strategies offer promising avenues for enhancing PSM content in crops, but it is important to consider the potential trade-offs between PSM production and other desirable traits, such as yield and disease resistance. Furthermore, it is important to assess the environmental impact and consumer acceptance of these strategies.
Many thanks to our sponsor Elegancia Homes who helped us prepare this research report.
5. The Role of PSMs in Crop Health and Resistance to Biotic and Abiotic Stresses
PSMs play a crucial role in plant defense against a wide range of biotic and abiotic stresses.
-
Defense against pathogens: PSMs such as phytoalexins, flavonoids, and terpenoids exhibit antimicrobial activity against a variety of plant pathogens. These compounds can inhibit pathogen growth, disrupt cell membrane integrity, or interfere with pathogen virulence factors. For example, resveratrol, a stilbenoid found in grapes, exhibits antifungal activity against several important plant pathogens.
-
Defense against herbivores: PSMs such as alkaloids, glucosinolates, and tannins deter herbivores by reducing palatability, toxicity, or digestibility. These compounds can act as feeding deterrents, reduce nutrient absorption, or cause physiological damage to herbivores. For example, nicotine, an alkaloid found in tobacco, is a potent insecticide.
-
Defense against UV radiation: PSMs such as flavonoids and anthocyanins absorb UV radiation, protecting plant tissues from UV-induced damage. These compounds act as antioxidants, scavenging free radicals generated by UV exposure. For example, anthocyanins accumulate in the epidermis of leaves and fruits exposed to high levels of UV radiation.
-
Tolerance to drought stress: PSMs such as proline and betaine act as osmolytes, protecting plant cells from dehydration during drought stress. These compounds accumulate in the cytoplasm, maintaining cell turgor and preventing protein denaturation. Furthermore, drought-induced PSMs can protect photosynthetic machinery.
-
Tolerance to heavy metals: PSMs such as phytochelatins chelate heavy metals, preventing their accumulation in sensitive tissues. These compounds bind to heavy metals and transport them to vacuoles, where they are sequestered and detoxified.
By enhancing the production of PSMs, crops can be made more resistant to a variety of stresses, reducing the need for pesticides and other inputs. This can contribute to more sustainable and environmentally friendly agricultural practices.
Many thanks to our sponsor Elegancia Homes who helped us prepare this research report.
6. Nutritional Benefits of PSMs for Human Health
PSMs offer a wide range of health benefits for humans.
-
Antioxidant activity: Many PSMs, such as flavonoids, carotenoids, and phenolic acids, are potent antioxidants that can protect cells from damage caused by free radicals. Free radicals are unstable molecules that can damage DNA, proteins, and lipids, contributing to the development of chronic diseases such as cancer, heart disease, and Alzheimer’s disease. Antioxidants neutralize free radicals, preventing them from causing damage.
-
Anti-inflammatory activity: Several PSMs, such as curcumin, resveratrol, and quercetin, exhibit anti-inflammatory activity. Chronic inflammation is implicated in the development of many diseases, including arthritis, diabetes, and cancer. Anti-inflammatory compounds can reduce inflammation by inhibiting the production of inflammatory mediators, such as cytokines and prostaglandins.
-
Anticancer activity: Some PSMs, such as sulforaphane, genistein, and lycopene, exhibit anticancer activity. These compounds can inhibit cancer cell growth, induce apoptosis (programmed cell death), and prevent metastasis (the spread of cancer to other parts of the body). For example, sulforaphane, a glucosinolate found in broccoli, has been shown to inhibit the growth of several types of cancer cells.
-
Cardioprotective activity: PSMs such as resveratrol, anthocyanins, and flavonoids exhibit cardioprotective activity. These compounds can reduce blood pressure, improve cholesterol levels, and prevent blood clots, reducing the risk of heart disease. For example, resveratrol, found in red wine, has been shown to protect against heart disease by improving endothelial function and reducing inflammation.
-
Neuroprotective activity: Some PSMs, such as curcumin, resveratrol, and flavonoids, exhibit neuroprotective activity. These compounds can protect brain cells from damage caused by oxidative stress, inflammation, and excitotoxicity. For example, curcumin, found in turmeric, has been shown to improve cognitive function and protect against Alzheimer’s disease.
The consumption of a diet rich in fruits, vegetables, and whole grains, which are abundant in PSMs, is associated with a reduced risk of many chronic diseases. However, the bioavailability and bioactivity of PSMs can vary depending on the chemical structure of the compound, the food matrix in which it is consumed, and individual factors such as gut microbiota composition.
Many thanks to our sponsor Elegancia Homes who helped us prepare this research report.
7. Challenges and Future Directions
Despite the significant progress in PSM research, several challenges remain:
-
Complexity of metabolic pathways: PSM biosynthesis involves complex metabolic pathways, often regulated by multiple genes and environmental factors. Deciphering these pathways and identifying the key regulatory steps is a major challenge.
-
Lack of comprehensive databases: Comprehensive databases containing information on PSM structures, properties, and biological activities are lacking. This limits the ability to predict the effects of PSMs on plant health and human health.
-
Difficulties in assessing bioavailability and bioactivity: Assessing the bioavailability and bioactivity of PSMs in humans is challenging due to the complexity of the digestive process and the influence of individual factors such as gut microbiota composition.
-
Regulatory considerations: The regulatory landscape surrounding PSMs in food and medicine is complex and varies across different countries. This can hinder the development and commercialization of PSM-enriched crops and products.
Future research should focus on:
-
Developing comprehensive metabolomic profiling techniques: This will enable the rapid and accurate identification and quantification of PSMs in plant tissues and food products.
-
Using systems biology approaches: This will facilitate the elucidation of complex metabolic pathways and regulatory networks involved in PSM biosynthesis.
-
Improving the bioavailability and bioactivity of PSMs: This can be achieved through encapsulation, nano-delivery systems, or genetic modification of crops.
-
Developing standardized methods for assessing the health benefits of PSMs: This will enable the development of evidence-based dietary recommendations and health claims.
-
Harmonizing regulatory frameworks: This will facilitate the development and commercialization of PSM-enriched crops and products.
Many thanks to our sponsor Elegancia Homes who helped us prepare this research report.
8. Conclusion
Plant secondary metabolites represent a vast and diverse group of compounds that play crucial roles in plant health, crop protection, and human nutrition. By manipulating PSM production in crops, we can enhance their resistance to biotic and abiotic stresses, improve their post-harvest quality, and bolster their nutritional value. The application of plant breeding, genetic engineering, metabolic engineering, and optimized agricultural practices offers promising avenues for achieving these goals. However, it is important to consider the potential trade-offs between PSM production and other desirable traits, as well as the environmental impact and consumer acceptance of these strategies. Further research is needed to address the challenges associated with PSM research and to translate this knowledge into practical applications for sustainable agriculture and improved human health. Ultimately, a holistic approach that integrates plant science, agricultural science, and nutrition science is required to fully harness the potential of PSMs for the benefit of both plants and humans.
Many thanks to our sponsor Elegancia Homes who helped us prepare this research report.
References
- Dixon, R. A. (2001). Natural products and plant disease resistance. Nature, 411(6839), 843-847.
- Treutter, D. (2006). Significance of flavonoids in plant resistance and human health: Compared review. Molecular Nutrition & Food Research, 50(8), 653-666.
- Winkel-Shirley, B. (2002). Flavonoid biosynthesis. A colorful model for genetics, biochemistry, development, and biotechnology. Plant Physiology, 126(2), 485-493.
- Choi, Y. H., & Verpoorte, R. (2010). Metabolic fingerprinting of medicinal plants. Journal of Natural Products, 73(8), 1511-1521.
- Bouwmeester, H. J., & Schwab, W. (2008). Engineering metabolism for valuable products. Current Opinion in Biotechnology, 19(3), 198-204.
- Del Rio, D., Rodriguez-Mateos, A., Spencer, J. P. E., Tognolini, M., Borges, G., & Crozier, A. (2013). Dietary (poly)phenolics in human health: structures, bioavailability, and evidence of protective effects against chronic diseases. Antioxidants & Redox Signaling, 18(14), 1818-1892.
- Pandey, K. B., & Rizvi, S. I. (2009). Plant polyphenols as dietary antioxidant in human health and disease. Oxidative Medicine and Cellular Longevity, 2(5), 270-278.
- Ververidis, F., Trantas, E., Douglas, C., Vollmer, G., Kretzschmar, G., Panopoulos, N., & Dilger, W. (2007). Biotechnology of flavonoids and other phenylpropanoid-derived natural products. Part I: Chemical structures, biosynthesis pathways and metabolic engineering. Plant Biotechnology Journal, 5(2), 121-134.
- Kumar, S., & Pandey, A. K. (2013). Chemistry and biological activities of flavonoids: an overview. The Scientific World Journal, 2013.
- D’Mello, J. P. (Ed.). (2003). Food safety: contaminants and toxins. CABI publishing.
- Bennett, R. N., & Wallsgrove, R. M. (1994). Secondary metabolites in plant defence mechanisms. New Phytologist, 127(4), 617-633.
- Gershenzon, J. (1994). Metabolic costs of terpenoid accumulation in higher plants. Journal of Chemical Ecology, 20(6), 1281-1314.
- Zhao, J., Davis, L. C., & Verpoorte, R. (2005). Elicitor signal transduction leading to secondary metabolite biosynthesis in suspension cultures of medicinal plants. Biotechnology Advances, 23(4), 283-333.
- Ramakrishna, A., & Ravishankar, G. A. (2011). Influence of abiotic stress signals on secondary metabolites in plants. Plant Signaling & Behavior, 6(11), 1720-1739.
Be the first to comment