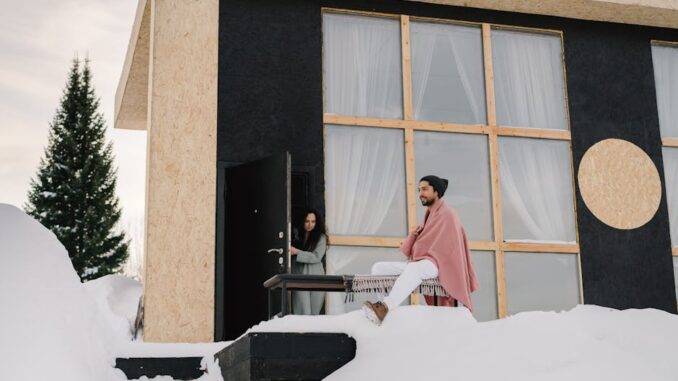
Advanced Energy Efficiency Strategies for Building Envelopes: Beyond Incremental Improvements
Abstract
Energy efficiency in buildings is paramount for mitigating climate change, reducing energy consumption, and improving occupant comfort. This report delves beyond conventional approaches like glazing upgrades and insulation, exploring advanced technologies and strategies for optimizing building envelope performance. We examine dynamic insulation systems, advanced glazing technologies, smart building integration, and the role of bio-based materials, focusing on their potential for significant energy savings and enhanced building resilience. A critical assessment of cost-effectiveness, long-term performance, and environmental impact is provided, along with recommendations for future research and development to facilitate widespread adoption of these innovative solutions.
1. Introduction
The imperative for energy efficiency in the built environment is driven by the dual challenges of escalating energy demand and the pressing need to reduce greenhouse gas emissions. The building envelope, comprising walls, roofs, windows, and foundations, plays a crucial role in determining a building’s energy performance. It acts as a barrier against heat transfer, moisture intrusion, and air leakage, directly impacting the heating, cooling, and ventilation loads required to maintain comfortable indoor conditions.
While traditional energy efficiency measures, such as improving insulation levels and upgrading to energy-efficient windows, have contributed to reducing energy consumption, they often fall short of achieving the deep decarbonization targets required to mitigate climate change. Furthermore, these incremental improvements may not adequately address the complex interplay of factors affecting building envelope performance, including dynamic environmental conditions, occupant behavior, and building design. This research report explores advanced strategies for building envelope optimization that go beyond conventional approaches, aiming to unlock significant energy savings and enhance building resilience.
The focus is on emerging technologies and innovative design approaches that can dramatically improve the thermal performance, airtightness, and overall energy efficiency of building envelopes. These strategies encompass dynamic insulation systems that adapt to changing environmental conditions, advanced glazing technologies with superior solar heat gain control, smart building integration for automated energy management, and the use of bio-based materials with low embodied energy. The report also includes a critical assessment of the cost-effectiveness, long-term performance, and environmental impact of these advanced solutions, along with recommendations for future research and development to accelerate their adoption.
2. Dynamic Insulation Systems
Dynamic insulation systems represent a paradigm shift from static insulation approaches by actively adapting their thermal resistance to changing environmental conditions. These systems typically employ air cavities within the building envelope, coupled with controllable airflow mechanisms, to modulate heat transfer based on external temperature, solar radiation, and occupant needs.
One prominent example is transpired solar collectors, which utilize perforated metal cladding to absorb solar energy and preheat air before it enters the building. By adjusting the airflow rate through the perforations, the amount of solar heat gain can be controlled, minimizing overheating in summer and maximizing heat recovery in winter. Computational Fluid Dynamics (CFD) modelling is essential for optimizing the design and performance of transpired solar collectors, taking into account factors such as perforation size, airflow rate, and cladding material properties.
Another type of dynamic insulation system involves the use of aerogel-filled cavities with variable ventilation. Aerogels are highly porous materials with exceptional thermal insulation properties. By varying the airflow through the aerogel-filled cavities, the effective thermal resistance of the building envelope can be dynamically adjusted. This approach is particularly effective in buildings with significant diurnal temperature variations, allowing for optimized energy performance throughout the day.
Further advancements include the integration of phase change materials (PCMs) within dynamic insulation systems. PCMs absorb and release heat as they undergo a phase transition (e.g., solid to liquid), providing thermal inertia and reducing temperature fluctuations. By incorporating PCMs into dynamic insulation systems, the building envelope can effectively store solar energy during the day and release it at night, reducing the reliance on active heating and cooling systems. The effectiveness of PCM integration hinges on selecting appropriate PCMs with suitable melting temperatures and thermal properties, as well as optimizing their placement within the building envelope. Research by Kuznik et al. (2011) demonstrated significant energy savings using PCM integrated walls compared to conventional insulation.
The primary challenge associated with dynamic insulation systems is the complexity of their design and control. Optimizing the airflow rates and PCM melting temperatures requires sophisticated modeling and control algorithms. Furthermore, the long-term performance and reliability of these systems must be carefully evaluated to ensure their economic viability.
3. Advanced Glazing Technologies
Windows are often the weakest link in the building envelope from an energy perspective, accounting for a significant portion of heat loss in winter and heat gain in summer. Advanced glazing technologies aim to mitigate these issues by improving the thermal resistance, solar heat gain coefficient (SHGC), and visible light transmittance of windows.
Low-emissivity (low-E) coatings are thin, transparent coatings applied to glass surfaces to reduce radiative heat transfer. These coatings selectively transmit visible light while reflecting infrared radiation, minimizing heat loss in winter and heat gain in summer. Different types of low-E coatings are available, with varying SHGC and visible light transmittance values, allowing for tailored performance based on climate and building orientation.
Gas-filled windows, which incorporate an inert gas such as argon or krypton between the glass panes, further enhance thermal resistance. These gases have lower thermal conductivity than air, reducing conductive heat transfer through the window assembly. Krypton, with its lower thermal conductivity, offers superior performance compared to argon but is more expensive.
Suspended particle devices (SPDs) represent a more advanced glazing technology that allows for dynamic control of light and heat transmission. SPDs consist of microscopic particles suspended in a liquid film between two glass panes. By applying an electric field, the particles align, allowing light to pass through. When the electric field is removed, the particles randomly orient, blocking light and heat. SPDs offer the potential for significant energy savings by dynamically adjusting the SHGC of windows based on solar radiation and occupant needs. Recent research by Baetens et al. (2010) highlight the potential energy saving with dynamic shading devices such as SPDs.
Vacuum insulated glazing (VIG) offers extremely high thermal resistance by creating a vacuum between two glass panes. This eliminates conductive and convective heat transfer, resulting in U-values comparable to those of well-insulated walls. VIG technology is particularly suitable for applications where space is limited, such as historic buildings or high-rise structures. However, VIG technology is still relatively expensive and requires careful manufacturing to maintain the vacuum seal.
Electrochromic windows are another type of dynamic glazing that changes its light transmittance in response to an applied voltage. These windows can be switched from a transparent state to a tinted or opaque state, allowing for dynamic control of solar heat gain and glare. Electrochromic windows offer a more subtle shading control compared to SPDs, making them suitable for applications where maintaining daylight levels is important. The durability and switching speed of electrochromic windows are key factors affecting their performance and cost-effectiveness.
4. Smart Building Integration and Automated Energy Management
The integration of advanced glazing technologies and dynamic insulation systems requires sophisticated control strategies to optimize their performance. Smart building technologies, including sensors, actuators, and control algorithms, enable automated energy management based on real-time environmental conditions, occupant behavior, and energy prices.
Building automation systems (BAS) can be used to monitor and control the performance of dynamic insulation systems, adjusting airflow rates and PCM melting temperatures based on weather forecasts and occupancy patterns. By integrating BAS with advanced glazing technologies, the SHGC of windows can be dynamically adjusted to minimize solar heat gain in summer and maximize solar heat gain in winter.
Occupancy sensors can be used to detect the presence of occupants in different zones of the building, allowing for targeted control of heating, cooling, and lighting systems. This can significantly reduce energy consumption in unoccupied areas. Advanced control algorithms, such as model predictive control (MPC), can be used to optimize the operation of building systems based on predicted future conditions. MPC algorithms take into account factors such as weather forecasts, occupancy patterns, and energy prices to minimize energy consumption while maintaining comfortable indoor conditions. Research by Afroz et al. (2016) shows the effectiveness of MPC for energy efficient building climate control.
The integration of smart building technologies with renewable energy sources, such as solar photovoltaic (PV) panels, can further enhance energy efficiency and reduce carbon emissions. Building energy management systems (BEMS) can be used to optimize the use of on-site renewable energy generation, storing excess energy in batteries for later use or exporting it to the grid. The integration of smart building technologies requires careful consideration of data security and privacy issues. It is essential to implement robust cybersecurity measures to protect building systems from unauthorized access and cyberattacks. Furthermore, it is important to ensure that occupant data is collected and used in a transparent and ethical manner, respecting privacy rights.
5. Bio-Based and Sustainable Building Materials
The environmental impact of building materials is a critical consideration in the pursuit of sustainable building design. Conventional building materials, such as concrete and steel, have high embodied energy and carbon footprints. Bio-based materials, such as wood, bamboo, hemp, and straw, offer a more sustainable alternative, with lower embodied energy and carbon sequestration potential.
Wood is a widely used bio-based building material with excellent structural properties and thermal insulation capabilities. Sustainable forestry practices ensure that wood is harvested in a responsible manner, minimizing environmental impact. Engineered wood products, such as cross-laminated timber (CLT) and glued laminated timber (glulam), offer increased strength and dimensional stability compared to solid wood, allowing for larger spans and more complex building designs. Research by Buchanan and Levine (1999) highlight the carbon benefits of using wood products in construction.
Hempcrete, a composite material made from hemp shivs (the woody core of the hemp plant), lime, and water, offers excellent thermal insulation and moisture regulation properties. Hempcrete is also highly breathable, allowing moisture to escape from the building envelope, preventing mold growth and improving indoor air quality.
Straw bale construction is another sustainable building technique that utilizes bales of straw as insulation and structural elements. Straw bales have excellent thermal insulation properties and are readily available in many regions. However, straw bale construction requires careful design and detailing to ensure proper moisture management and structural stability.
Mycelium-based materials, grown from the root structure of fungi, are an emerging class of bio-based materials with potential applications in insulation and packaging. Mycelium can be grown on agricultural waste products, such as sawdust and straw, providing a sustainable alternative to conventional insulation materials. Mycelium-based materials are biodegradable and compostable, reducing waste and minimizing environmental impact. However, the long-term durability and fire resistance of mycelium-based materials are still under investigation.
The use of bio-based building materials can significantly reduce the embodied energy and carbon footprint of buildings. However, it is essential to consider the entire life cycle of these materials, including their sourcing, manufacturing, transportation, and disposal. Life cycle assessment (LCA) is a valuable tool for evaluating the environmental impact of different building materials and comparing their sustainability performance.
6. Cost-Benefit Analysis and Economic Viability
The economic viability of advanced energy efficiency strategies is a critical factor in their widespread adoption. A comprehensive cost-benefit analysis should consider the initial investment costs, energy savings, maintenance costs, and potential environmental benefits. The lifecycle cost analysis (LCCA) is a useful technique for evaluating the long-term economic performance of different building envelope options, taking into account the time value of money.
Dynamic insulation systems and advanced glazing technologies typically have higher initial investment costs compared to conventional insulation and window systems. However, the potential energy savings can offset these higher costs over the lifetime of the building. The payback period, which is the time required to recoup the initial investment through energy savings, is a key metric for evaluating the economic viability of these technologies.
Government incentives and rebates can play a significant role in promoting the adoption of advanced energy efficiency technologies. Tax credits, grants, and low-interest loans can reduce the upfront costs, making these technologies more affordable for building owners. Performance-based incentives, which reward building owners for achieving specific energy savings targets, can also encourage investment in energy efficiency measures.
The cost-effectiveness of smart building integration depends on the complexity of the system and the potential for energy savings. While the initial investment in sensors, actuators, and control algorithms can be significant, the potential for automated energy management and optimized building performance can result in substantial long-term savings. The integration of smart building technologies can also improve occupant comfort and productivity, leading to additional economic benefits.
The economic viability of bio-based building materials depends on their availability, processing costs, and performance characteristics. While some bio-based materials, such as wood, are readily available and cost-competitive, others, such as hempcrete and mycelium-based materials, are still in the early stages of commercialization and may be more expensive. However, as demand for these materials increases and production processes become more efficient, their costs are likely to decrease, making them more economically viable.
7. Future Research and Development
Further research and development are needed to accelerate the adoption of advanced energy efficiency strategies for building envelopes. Key areas for future research include:
- Development of more cost-effective dynamic insulation systems: Research should focus on simplifying the design and control of dynamic insulation systems, reducing their manufacturing costs, and improving their long-term reliability.
- Improvement of the performance and durability of advanced glazing technologies: Research should focus on developing new low-E coatings with higher visible light transmittance and lower SHGC, improving the durability of electrochromic windows, and reducing the cost of vacuum insulated glazing.
- Development of more sophisticated control algorithms for smart building integration: Research should focus on developing MPC algorithms that can adapt to changing environmental conditions, occupant behavior, and energy prices in real time. The research should also focus on cybersecurity aspect of smart building technologies.
- Further investigation of the performance and durability of bio-based building materials: Research should focus on evaluating the long-term performance of bio-based building materials in different climates and applications, developing improved fire-resistant treatments, and optimizing their manufacturing processes.
- Development of standardized testing methods for advanced energy efficiency technologies: Standardized testing methods are needed to accurately evaluate the performance of dynamic insulation systems, advanced glazing technologies, and bio-based building materials, providing reliable data for designers and policymakers.
- Life cycle assessment (LCA) of advanced building envelope technologies: Detailed LCA studies are needed to accurately assess the environmental impact of different building envelope options, considering their embodied energy, carbon footprint, and potential for resource depletion.
8. Conclusion
Advanced energy efficiency strategies for building envelopes offer significant potential for reducing energy consumption, mitigating climate change, and enhancing building resilience. Dynamic insulation systems, advanced glazing technologies, smart building integration, and bio-based materials represent innovative approaches that can dramatically improve the thermal performance, airtightness, and overall energy efficiency of buildings. While these technologies often have higher initial investment costs compared to conventional approaches, the potential energy savings and environmental benefits can outweigh these costs over the lifetime of the building. Further research and development are needed to reduce the costs, improve the performance, and standardize the testing methods for these advanced technologies, facilitating their widespread adoption. Government incentives, performance-based rewards, and clear industry standards can further encourage the implementation of these advanced building envelope techniques.
References
- Afroz, Z., Khan, M. R., & Mansur, M. (2016). Model predictive control for energy efficient building climate control: A review. Renewable and Sustainable Energy Reviews, 60, 1349-1362.
- Baetens, R., Jelle, B. P., & Gustavsen, A. (2010). Properties, requirements and possibilities of smart windows for dynamic daylight and solar energy control in buildings: A state-of-the-art review. Solar Energy Materials and Solar Cells, 94(2), 87-105.
- Buchanan, A. H., & Levine, S. B. (1999). Wood as a structural material. A material for sustainable construction. Journal of Sustainable Forestry, 9(1-2), 9-60.
- Kuznik, F., Virgone, N., & Johannes, L. (2011). Characterisation of thermal behaviour of building materials incorporating PCM. Renewable Energy, 36(1), 408-416.
The discussion of dynamic insulation systems is compelling, particularly the use of phase change materials. How might these systems be adapted for retrofitting existing buildings, considering the challenges of integrating them into existing wall structures?
Dynamic insulation systems adapting to environmental conditions? Sounds like buildings are finally getting a bit of common sense! Next step: buildings that can order their own coffee based on occupant mood.
Given the advancements in dynamic glazing technologies like SPDs and electrochromic windows, what are the primary barriers to their widespread adoption in residential construction beyond initial cost?
Considering the potential of bio-based materials like mycelium for insulation, how do current building codes address or hinder their implementation, particularly regarding fire resistance and structural integrity?
Bio-based materials are intriguing! Imagine buildings literally growing into energy efficiency. Wonder if we’ll see homes cultivated from mycelium, complete with self-repairing insulation?
The discussion of smart building integration highlights the potential for significant energy savings through automated energy management. Expanding on this, how can AI and machine learning be further leveraged to predict and optimize building energy consumption in real-time based on occupancy patterns and external factors?
Smart building integration? Sounds like Skynet, but for HVAC. Perhaps we can train our buildings to negotiate energy rates with the grid, or at least argue with the thermostat on our behalf?
The mention of integrating renewable energy sources with smart building technologies raises interesting possibilities. How can blockchain technology further enhance building energy management by enabling peer-to-peer energy trading within a community microgrid?
Buildings that order their own coffee? I’m holding out for self-aware buildings that can negotiate property taxes and maybe even handle HOA meetings. Talk about advanced energy savings – imagine the reduced stress on residents!
Given the embodied energy benefits of bio-based materials, what innovative financial incentives or policy changes could encourage developers to prioritize their use over conventional materials despite potentially higher upfront costs?