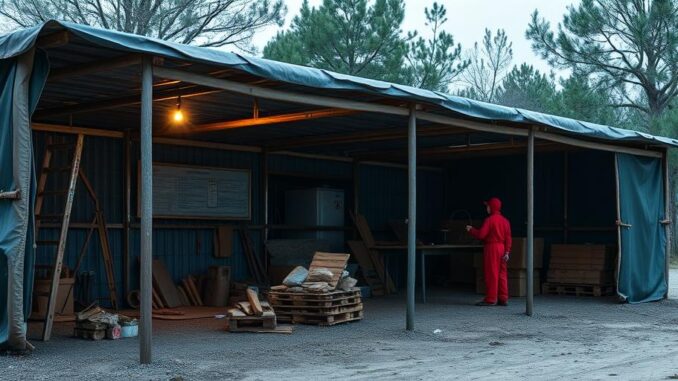
Abstract
This research report examines advanced material selection strategies for high-performance enclosure systems, moving beyond traditional timber versus uPVC debates. It employs a multi-criteria analysis framework, evaluating materials based on mechanical properties, thermal performance, environmental impact (embodied carbon and life cycle assessment), aesthetic considerations, and economic feasibility. The study explores established materials like engineered wood products (e.g., cross-laminated timber – CLT, glued laminated timber – Glulam), high-performance concrete (HPC), structural insulated panels (SIPs), and advanced polymers, juxtaposing them against emerging technologies such as bio-based composites, aerogels, and vacuum insulated panels (VIPs). Furthermore, it investigates the integration of smart materials for adaptive enclosure systems. The report concludes by proposing a decision-making framework incorporating weighted criteria and performance indices to guide material selection based on specific project requirements and sustainability targets, addressing the complex interplay between performance, cost, and environmental responsibility in modern enclosure design.
Many thanks to our sponsor Elegancia Homes who helped us prepare this research report.
1. Introduction
Enclosure systems, encompassing walls, roofs, and fenestration, represent a critical interface between the built environment and the external climate. Their performance directly impacts energy consumption, indoor environmental quality, and the overall longevity and sustainability of a building. Traditionally, material selection for these systems has been driven by factors such as cost and local availability, often leading to suboptimal performance in terms of thermal efficiency, durability, and environmental impact. This report challenges this conventional approach, advocating for a more holistic and performance-driven material selection process. The focus shifts from solely considering initial costs to evaluating the life-cycle performance of materials, taking into account factors like embodied carbon, operational energy savings, maintenance requirements, and end-of-life scenarios. The increasing demand for energy-efficient and sustainable buildings necessitates a comprehensive understanding of material properties and their suitability for specific applications within enclosure systems. Furthermore, the report acknowledges the growing significance of aesthetic considerations and the potential for innovative materials to enhance architectural design and create distinctive building identities. This research aims to provide a robust framework for navigating the complexities of material selection in the context of high-performance enclosure systems, facilitating informed decisions that align with both performance objectives and sustainability goals.
Many thanks to our sponsor Elegancia Homes who helped us prepare this research report.
2. Evaluation Criteria for Enclosure Materials
A comprehensive evaluation of materials for enclosure systems requires a multifaceted approach considering various performance parameters. This section details the key criteria used for assessing material suitability.
2.1 Mechanical Properties
Mechanical properties dictate a material’s structural integrity and ability to withstand loads and stresses. Key considerations include:
- Tensile Strength: The material’s resistance to being pulled apart.
- Compressive Strength: The material’s resistance to being crushed.
- Flexural Strength: The material’s resistance to bending.
- Shear Strength: The material’s resistance to forces acting parallel to a surface.
- Elastic Modulus (Young’s Modulus): A measure of stiffness, indicating how much a material deforms under stress.
- Hardness: Resistance to indentation.
- Impact Resistance: Ability to withstand sudden shocks and impacts.
- Creep Resistance: Ability to resist deformation under sustained load over time. This is particularly relevant for materials like polymers used in structural applications.
- Fatigue Resistance: The ability to withstand repeated cycles of stress. This is essential for components exposed to wind loads or other dynamic forces.
The relevance of each of these properties varies depending on the specific application within the enclosure system. For example, compressive strength is critical for load-bearing walls, while flexural strength is essential for roof elements. Materials that exhibit high strength-to-weight ratios are particularly desirable, as they minimize the overall weight of the structure and reduce transportation costs.
2.2 Thermal Performance
Thermal performance is paramount in achieving energy efficiency and maintaining indoor comfort. Key metrics include:
- Thermal Conductivity (k-value): The measure of a material’s ability to conduct heat. Lower k-values indicate better insulation properties.
- Thermal Resistance (R-value): The measure of a material’s resistance to heat flow. Higher R-values indicate better insulation properties. This is dependent on material thickness.
- Thermal Transmittance (U-value): The overall heat transfer coefficient through a building element (e.g., a wall or window). Lower U-values indicate better insulation performance of the entire assembly. This is affected by the R-values of all materials used and any air gaps.
- Specific Heat Capacity (Cp): The amount of heat required to raise the temperature of a unit mass of a material by one degree Celsius. Materials with high specific heat capacity can store more heat, contributing to thermal mass effects.
- Thermal Inertia: A measure of a material’s ability to resist changes in temperature. High thermal inertia can dampen temperature fluctuations and improve indoor comfort.
- Solar Heat Gain Coefficient (SHGC): The fraction of solar radiation that enters a building through a window or glazing system. Lower SHGC values are desirable in hot climates to reduce cooling loads.
- Emissivity: The measure of a material’s ability to radiate heat. Low emissivity materials are effective at reducing radiative heat transfer.
The choice of insulation materials and their placement within the enclosure system is crucial for optimizing thermal performance. The use of thermal bridging mitigation strategies is also essential to minimize heat loss through structural elements.
2.3 Environmental Impact
Environmental impact is increasingly important in sustainable building design. Key considerations include:
- Embodied Carbon: The total greenhouse gas emissions associated with the extraction, processing, manufacturing, transportation, and installation of a material. This includes the CO2 associated with material production and its end-of-life disposal.
- Life Cycle Assessment (LCA): A comprehensive analysis of the environmental impacts of a material throughout its entire life cycle, from cradle to grave. This includes energy consumption, water usage, air and water pollution, and waste generation.
- Recyclability and Reusability: The ability of a material to be recycled or reused at the end of its life. Materials with high recyclability reduce the demand for virgin resources and minimize waste.
- Renewable Resource Content: The proportion of a material derived from renewable resources, such as wood or plant-based fibers. The use of renewable materials reduces reliance on fossil fuels.
- Toxicity: The potential for a material to release harmful chemicals or pollutants into the environment during its production, use, or disposal. Low-toxicity materials are preferred to minimize health risks.
- Water Usage: The amount of water consumed during the extraction, processing, and manufacturing of a material. Water scarcity is a growing concern, making water efficiency a crucial consideration.
- Biodegradability: The ability of a material to decompose naturally in the environment. Biodegradable materials can reduce landfill waste and contribute to a circular economy.
Selecting materials with low embodied carbon, high recyclability, and renewable resource content is essential for minimizing the environmental footprint of enclosure systems. Life cycle assessments provide a valuable tool for comparing the environmental performance of different material options.
2.4 Aesthetic Considerations
Aesthetic considerations are crucial for creating visually appealing and harmonious buildings. Key aspects include:
- Color and Texture: The visual appearance of a material, which can influence the overall character and atmosphere of a building.
- Formability and Flexibility: The ability of a material to be shaped and molded into complex forms, allowing for greater design freedom.
- Surface Finish: The texture and reflectivity of a material’s surface, which can affect its light-reflecting properties and overall visual appeal.
- Compatibility with Other Materials: The ability of a material to seamlessly integrate with other building materials, creating a cohesive and aesthetically pleasing design.
- Longevity and Durability of Appearance: The ability of a material to retain its original appearance over time, resisting fading, discoloration, and other forms of degradation.
- Architectural Style: The compatibility of a material with the overall architectural style of the building. Certain materials are more suited to specific styles, such as modern, traditional, or contemporary.
The aesthetic qualities of enclosure materials can significantly impact the perceived value and appeal of a building. Selecting materials that complement the architectural design and enhance the overall visual experience is essential.
2.5 Economic Feasibility
Economic feasibility is a critical factor in material selection. Key considerations include:
- Initial Cost: The purchase price of the material, including transportation and installation costs.
- Life Cycle Cost: The total cost of a material over its entire lifespan, including initial cost, maintenance costs, repair costs, and replacement costs.
- Energy Savings: The potential for a material to reduce energy consumption and lower utility bills.
- Maintenance Requirements: The frequency and cost of maintenance required to keep a material in good condition.
- Durability and Longevity: The expected lifespan of a material, which can impact replacement costs and overall life cycle cost.
- Installation Costs: The labor and equipment costs associated with installing a material. Some materials are easier and less expensive to install than others.
- Salvage Value: The value of a material at the end of its useful life, which can offset disposal costs.
A thorough economic analysis is essential for identifying the most cost-effective material options over the long term. Life cycle cost analysis provides a valuable tool for comparing the total cost of ownership of different materials.
Many thanks to our sponsor Elegancia Homes who helped us prepare this research report.
3. Established Enclosure Materials: Properties and Applications
This section examines established materials commonly used in enclosure systems, highlighting their properties, advantages, and disadvantages.
3.1 Engineered Wood Products (EWP)
Engineered wood products, such as CLT and Glulam, offer a sustainable alternative to concrete and steel in structural applications.
- Cross-Laminated Timber (CLT): CLT is a massive timber product made by layering and gluing together multiple layers of lumber, with each layer oriented perpendicular to the adjacent layers. This cross-lamination provides excellent dimensional stability and load-bearing capacity. CLT offers high strength-to-weight ratio, good thermal insulation, and carbon sequestration benefits. However, it is susceptible to moisture damage if not properly protected and can be more expensive than traditional wood framing. Fire resistance can be achieved through charring layer design.
- Glued Laminated Timber (Glulam): Glulam is made by gluing together individual pieces of lumber to create large, structural members. Glulam offers high strength, design flexibility, and aesthetic appeal. It is suitable for long-span beams and columns. Similar to CLT, Glulam requires protection from moisture and can be susceptible to fire damage if not properly treated.
EWPs are gaining popularity in sustainable construction due to their renewable resource content and carbon sequestration potential. They are particularly well-suited for prefabricated building systems, allowing for faster and more efficient construction.
3.2 High-Performance Concrete (HPC)
High-performance concrete (HPC) is a type of concrete that is specifically designed to exhibit enhanced durability, strength, and other desirable properties compared to conventional concrete.
- Properties: HPC typically has a higher compressive strength, lower permeability, and improved resistance to cracking and chemical attack. It often incorporates supplementary cementitious materials (SCMs) such as fly ash, slag, or silica fume to enhance its performance and reduce its environmental impact. HPC mixes often use superplasticizers to reduce the water to cement ratio, leading to high density.
- Applications: HPC is used in a wide range of applications, including bridges, high-rise buildings, and pavements. In enclosure systems, HPC can be used for load-bearing walls, cladding panels, and precast concrete elements.
While HPC offers improved durability and performance, it still has a relatively high embodied carbon footprint compared to other materials. The use of SCMs can help to reduce the environmental impact of HPC.
3.3 Structural Insulated Panels (SIPs)
Structural insulated panels (SIPs) are composite panels consisting of a core of rigid insulation (typically expanded polystyrene (EPS), extruded polystyrene (XPS), or polyurethane (PUR)) sandwiched between two structural facings (typically oriented strand board (OSB) or plywood).
- Properties: SIPs offer excellent thermal insulation, high structural strength, and airtightness. They can be used for walls, roofs, and floors. SIPs provide a fast and efficient construction method, reducing on-site labor and construction time.
- Applications: SIPs are commonly used in residential and commercial buildings, particularly in high-performance and energy-efficient construction. Their inherent insulation minimizes thermal bridging and reduces energy consumption.
While SIPs offer many advantages, the environmental impact of the insulation core and the facing materials should be considered. Some insulation materials, such as XPS and PUR, have high global warming potential. The use of bio-based or recycled insulation materials can help to reduce the environmental impact of SIPs.
3.4 Advanced Polymers
Advanced polymers, such as fiber-reinforced polymers (FRPs) and high-performance plastics, are increasingly used in enclosure systems due to their high strength-to-weight ratio, corrosion resistance, and design flexibility.
- Fiber-Reinforced Polymers (FRPs): FRPs consist of reinforcing fibers (e.g., carbon fiber, glass fiber, aramid fiber) embedded in a polymer matrix (e.g., epoxy resin, polyester resin). FRPs offer high strength, stiffness, and durability. They are used in a variety of applications, including structural reinforcement, cladding panels, and window frames.
- High-Performance Plastics: High-performance plastics, such as polycarbonate and acrylic, offer excellent impact resistance, weatherability, and optical clarity. They are used in glazing systems, skylights, and cladding panels.
While advanced polymers offer many advantages, their environmental impact is a concern. Most polymers are derived from fossil fuels and have a high embodied carbon footprint. Research is ongoing to develop bio-based and recycled polymers for use in building applications.
Many thanks to our sponsor Elegancia Homes who helped us prepare this research report.
4. Emerging Materials and Technologies
This section explores emerging materials and technologies that hold promise for enhancing the performance and sustainability of enclosure systems.
4.1 Bio-Based Composites
Bio-based composites are materials made from natural fibers (e.g., hemp, flax, jute) and bio-based resins (e.g., polylactic acid (PLA), epoxy resins derived from vegetable oils).
- Properties: Bio-based composites offer a renewable and sustainable alternative to conventional composites. They have good strength-to-weight ratio, thermal insulation, and acoustic properties. They also have a lower embodied carbon footprint than fossil fuel-based materials.
- Applications: Bio-based composites can be used for cladding panels, insulation, and structural components. They are particularly well-suited for applications where aesthetics and sustainability are important considerations.
However, the durability and long-term performance of bio-based composites are still being researched. Concerns include moisture resistance, fire resistance, and degradation over time. Further research and development are needed to improve the performance and reliability of these materials.
4.2 Aerogels
Aerogels are highly porous, solid materials with extremely low density and thermal conductivity.
- Properties: Aerogels offer exceptional thermal insulation properties, making them ideal for use in high-performance insulation systems. They also have good acoustic properties and are relatively lightweight.
- Applications: Aerogels can be used in insulation panels, glazing systems, and other building components. They are particularly well-suited for applications where space is limited and high thermal performance is required.
The cost of aerogels is currently relatively high, which limits their widespread adoption. However, as production volumes increase and manufacturing processes improve, the cost of aerogels is expected to decrease. Research is also ongoing to develop more durable and water-resistant aerogels.
4.3 Vacuum Insulated Panels (VIPs)
Vacuum insulated panels (VIPs) consist of a rigid core material (e.g., silica, fiberglass) encased in a gas-tight envelope that is evacuated to create a vacuum.
- Properties: VIPs offer extremely high thermal resistance, significantly outperforming conventional insulation materials. They are also relatively thin and lightweight.
- Applications: VIPs can be used in walls, roofs, and floors where space is limited and high thermal performance is required. They are particularly well-suited for retrofitting existing buildings to improve energy efficiency.
The cost of VIPs is currently relatively high, and they are susceptible to damage if the vacuum seal is compromised. However, ongoing research is focused on improving the durability and reducing the cost of VIPs.
4.4 Smart Materials for Adaptive Enclosure Systems
Smart materials are materials that can change their properties in response to external stimuli, such as temperature, light, or stress.
- Thermochromic Materials: Thermochromic materials change color in response to temperature changes. They can be used in glazing systems to control solar heat gain and reduce cooling loads.
- Photochromic Materials: Photochromic materials change color in response to light intensity. They can be used in glazing systems to reduce glare and improve visual comfort.
- Shape Memory Alloys (SMAs): SMAs are metals that can return to their original shape after being deformed. They can be used in adaptive shading devices and other building components that respond to changes in environmental conditions.
Smart materials offer the potential to create more dynamic and responsive enclosure systems that adapt to changing environmental conditions and optimize building performance. However, the cost and durability of smart materials are still challenges to their widespread adoption.
Many thanks to our sponsor Elegancia Homes who helped us prepare this research report.
5. Multi-Criteria Decision-Making Framework
The selection of enclosure materials is a complex process that requires careful consideration of multiple criteria. A multi-criteria decision-making (MCDM) framework can help to facilitate this process by providing a structured approach to evaluating and comparing different material options. The following steps are involved in developing an MCDM framework for enclosure material selection:
- Define Objectives: Clearly define the objectives of the enclosure system, such as minimizing energy consumption, maximizing indoor comfort, reducing environmental impact, and achieving specific aesthetic goals.
- Identify Criteria: Identify the relevant criteria for evaluating material options, based on the objectives. These criteria should include mechanical properties, thermal performance, environmental impact, aesthetic considerations, and economic feasibility.
- Assign Weights to Criteria: Assign weights to each criterion based on its relative importance. The weights should reflect the priorities of the project stakeholders.
- Evaluate Material Options: Evaluate each material option based on each criterion. This can be done using quantitative data (e.g., thermal conductivity, embodied carbon) or qualitative assessments (e.g., aesthetic appeal, ease of maintenance).
- Calculate Performance Indices: Calculate a performance index for each material option by multiplying the value of each criterion by its corresponding weight and summing the results. The performance index represents the overall performance of the material option based on the weighted criteria.
- Compare Material Options: Compare the performance indices of the different material options. The material option with the highest performance index is considered the best choice based on the MCDM framework.
- Sensitivity Analysis: Perform a sensitivity analysis to assess the impact of changes in the weights of the criteria on the ranking of the material options. This can help to identify the most critical criteria and to understand the robustness of the decision.
This framework can be implemented using various MCDM methods, such as the Analytical Hierarchy Process (AHP), the Technique for Order of Preference by Similarity to Ideal Solution (TOPSIS), or the Multi-Attribute Utility Theory (MAUT). The choice of method depends on the specific requirements of the project and the available data.
Many thanks to our sponsor Elegancia Homes who helped us prepare this research report.
6. Conclusion
Selecting appropriate materials for high-performance enclosure systems requires a detailed understanding of material properties, performance characteristics, and environmental impacts. This report has presented a multi-criteria analysis framework that considers mechanical performance, thermal efficiency, environmental sustainability, aesthetic appeal, and economic feasibility. By carefully evaluating materials based on these criteria, designers and engineers can make informed decisions that optimize building performance and minimize environmental impact. Emerging materials and technologies, such as bio-based composites, aerogels, and smart materials, offer exciting opportunities to further enhance the performance and sustainability of enclosure systems. However, further research and development are needed to address challenges related to cost, durability, and long-term performance. The continued adoption of life cycle assessment and multi-criteria decision-making frameworks will be crucial for promoting sustainable material selection practices and creating more energy-efficient and environmentally responsible buildings.
Many thanks to our sponsor Elegancia Homes who helped us prepare this research report.
References
- Asdrubali, F., Baldinelli, G., & Lunghi, P. (2015). Life cycle assessment in the construction sector: Comparing innovative and traditional solutions. Energy and Buildings, 107, 383-394.
- Pomponi, F., & Moncaster, A. (2016). Embodied carbon for buildings: A review. Renewable and Sustainable Energy Reviews, 65, 483-494.
- Ritchie, H., & Roser, M. (2020). CO₂ and Greenhouse Gas Emissions. Published online at OurWorldInData.org. Retrieved from: ‘https://ourworldindata.org/co2-and-other-greenhouse-gas-emissions’
- ISO 14040:2006, Environmental management — Life cycle assessment — Principles and framework
- European Commission. (2020). A European Green Deal. Retrieved from: ‘https://ec.europa.eu/info/strategy/priorities-2019-2024/european-green-deal_en’
- Myszka, J., & Chybinski, R. (2019). Multi-criteria decision support system for material selection in building envelopes. Buildings, 9(4), 91.
- Passipoularidis, V., & Bakos, G. C. (2018). Sustainable materials selection for building envelopes: A multi-criteria decision-making approach. Sustainability, 10(8), 2840.
- Karagkounis, V., Musa, S., Tikka, E., & Voulvoulis, N. (2020). A review of multi-criteria decision making methods for sustainable building design. Sustainable Cities and Society, 61, 102319.
- Zhang, X., & Wang, Z. (2017). Life cycle assessment of cross laminated timber building. Journal of Cleaner Production, 141, 108-117.
- Deru, M., Field, K., Frank, S., Pless, J., Torcellini, P., Griffith, B., … & Benne, K. (2011). High-performance building definition (No. NREL/TP-550-49767). National Renewable Energy Lab.(NREL), Golden, CO (United States).
- IEA. (2013). Transition to Sustainable Buildings: Strategies and Opportunities to 2050.
- Asdrubali, F., Piselli, C., & Baldinelli, G. (2013). A review of strategies for energy performance improvement in existing buildings. Renewable and Sustainable Energy Reviews, 27, 378-392.
- Holliday, J., & Barlow, C. Y. (2018). Sustainable construction. Routledge.
- ASTM E2129-19, Standard Practice for Data Collection for Sustainability Assessment of Building Products.
The report highlights the potential of smart materials like thermochromics in glazing. Could you elaborate on any practical limitations in their application regarding long-term performance and cost-effectiveness compared to static shading solutions?
The report mentions Vacuum Insulated Panels’ (VIPs) susceptibility to damage. What advancements are being made to enhance their structural integrity and resistance to puncture, especially considering their thin profile in retrofit applications?
This report rightly emphasizes the importance of aesthetic considerations in material selection. How can we better quantify the qualitative aspects of aesthetics to integrate them more effectively into the decision-making framework, ensuring designs are both functional and visually appealing?
Regarding embodied carbon, how does the framework account for biogenic carbon sequestration in materials like CLT, and are there standardized methodologies used to ensure accurate and consistent carbon accounting across different material types?
Given the complexities of LCA, how can the proposed MCDM framework best accommodate uncertainties and variations in environmental impact data across different geographical contexts and manufacturing processes?
Given the framework’s emphasis on life cycle costing, how does it address the potential for fluctuating energy prices and their subsequent impact on the long-term economic viability of different enclosure material choices?
Considering the report’s discussion of high-performance concrete, how do variations in local material sourcing (aggregates, cement replacements) affect its embodied carbon footprint, and how is this variability best integrated into the MCDM framework for material selection?
Considering the discussion of smart materials, how might the integration of sensors and IoT devices within enclosure systems contribute to a more dynamic and responsive building envelope, optimizing energy performance in real-time?