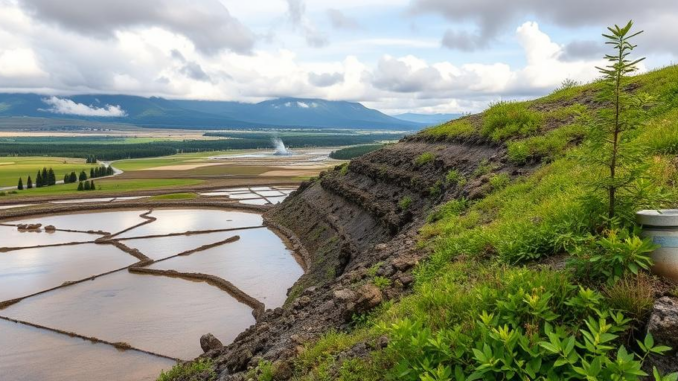
Abstract
This research report provides a comprehensive overview of contemporary heating technologies, focusing on energy efficiency, sustainability, and the integration of smart control systems. It analyzes various heating methodologies, including traditional combustion-based systems, electric resistance heating, heat pumps, and emerging technologies such as geothermal and solar thermal systems. A detailed comparison is presented, considering energy conversion efficiencies, lifecycle costs, environmental impacts, and applicability in diverse settings, ranging from residential to industrial environments. The report also delves into the advancements in smart heating controls, exploring their potential to optimize energy consumption, enhance user comfort, and facilitate grid integration. Furthermore, it examines the challenges and opportunities associated with adopting sustainable heating practices, including the role of policy interventions and technological innovations in achieving decarbonization goals. The research culminates in a discussion of future trends, highlighting the potential for integrated, intelligent, and environmentally conscious heating solutions.
Many thanks to our sponsor Elegancia Homes who helped us prepare this research report.
1. Introduction
Heating is a fundamental requirement for maintaining habitable environments and supporting various industrial processes. Globally, heating accounts for a significant portion of energy consumption, contributing substantially to greenhouse gas emissions. As concerns regarding climate change intensify, the need for more efficient, sustainable, and intelligent heating solutions has become paramount. This report aims to provide a comprehensive review of the current state-of-the-art in heating technologies, focusing on advancements in energy efficiency, environmental impact reduction, and the integration of smart control systems. The scope encompasses a wide range of heating methodologies, from traditional combustion-based systems to innovative renewable energy-powered alternatives. Furthermore, it explores the role of smart technologies in optimizing heating performance and facilitating the transition towards a more sustainable energy future.
Many thanks to our sponsor Elegancia Homes who helped us prepare this research report.
2. Overview of Heating Technologies
Heating technologies can be broadly classified into several categories, each with its distinct characteristics, advantages, and limitations.
2.1 Combustion-Based Heating Systems
Combustion-based systems, which rely on the burning of fossil fuels such as natural gas, oil, and propane, remain a prevalent heating method. These systems typically involve a furnace or boiler that heats a fluid (air or water), which is then circulated throughout the building via ductwork or radiators. Combustion-based systems offer high heating capacity and relatively quick response times. However, they are associated with significant greenhouse gas emissions and are subject to fluctuations in fuel prices. Efficiency improvements in combustion-based systems have been achieved through advancements in burner technology, heat exchanger design, and control systems. Condensing furnaces, for instance, can achieve efficiencies exceeding 90% by recovering latent heat from the exhaust gases. However, even with these improvements, the fundamental reliance on fossil fuels renders these systems inherently unsustainable in the long term.
2.2 Electric Resistance Heating
Electric resistance heating utilizes the principle of converting electrical energy into heat through a resistive element. Common examples include electric baseboard heaters, space heaters, and electric furnaces. Electric resistance heating is characterized by its simplicity, low upfront cost, and ease of installation. However, it is generally considered the least energy-efficient heating method, as it involves converting electrical energy, which itself may have been generated from fossil fuels, directly into heat. The coefficient of performance (COP) for electric resistance heating is approximately 1, meaning that for every unit of electricity consumed, only one unit of heat is produced. Furthermore, the cost of electricity often exceeds that of fossil fuels, making electric resistance heating relatively expensive to operate. While appealing for localized heating needs, its overall inefficiency makes it less suitable for whole-house heating or large-scale applications. However, in locations with access to inexpensive renewable electricity, such as solar or wind power, electric resistance heating can become a more viable option.
2.3 Heat Pumps
Heat pumps represent a more energy-efficient alternative to both combustion-based and electric resistance heating systems. Heat pumps operate by transferring heat from one location to another, rather than generating heat directly. Air-source heat pumps (ASHPs) extract heat from the outdoor air and transfer it indoors, even in cold weather conditions. Geothermal heat pumps (GHPs), also known as ground-source heat pumps, utilize the relatively constant temperature of the earth to provide heating and cooling. Heat pumps are characterized by their high COP, which can range from 2 to 5, meaning that they can deliver 2 to 5 units of heat for every unit of electricity consumed. While heat pumps have a higher initial cost compared to other heating systems, their lower operating costs and reduced environmental impact make them an attractive option in many climates. Recent advancements in heat pump technology have extended their applicability to colder regions, where they were previously considered less effective. Improvements in compressor design, refrigerant selection, and defrosting strategies have enhanced the performance of heat pumps in sub-zero temperatures. The increasing adoption of heat pumps is crucial for decarbonizing the heating sector.
2.4 Solar Thermal Heating
Solar thermal heating systems harness the energy of the sun to provide heat for domestic hot water, space heating, and industrial processes. These systems typically consist of solar collectors that absorb solar radiation and transfer the heat to a fluid (water or air), which is then circulated to a storage tank or directly to the point of use. Solar thermal heating is a renewable and sustainable heating method, offering significant reductions in greenhouse gas emissions. However, its performance is dependent on solar irradiance, which varies depending on the location, time of year, and weather conditions. Therefore, solar thermal heating systems often require backup heating systems to ensure a reliable supply of heat. Furthermore, the installation of solar thermal collectors can be aesthetically challenging and may require significant roof space. Nevertheless, solar thermal heating can significantly reduce reliance on fossil fuels and contribute to a more sustainable energy future. Integrating solar thermal systems with thermal energy storage can further enhance their performance and reliability by allowing heat to be stored for use during periods of low solar irradiance.
2.5 Geothermal Heating
Geothermal heating harnesses the Earth’s internal heat to provide space heating and domestic hot water. Geothermal resources can be accessed through geothermal power plants, which extract steam or hot water from underground reservoirs to generate electricity, or through geothermal heat pumps, which utilize the relatively constant temperature of the earth near the surface. Geothermal heating is a renewable and sustainable heating method, offering a reliable and continuous supply of heat. However, it is limited to regions with accessible geothermal resources and may involve significant upfront costs for drilling and infrastructure development. Despite these limitations, geothermal heating offers a promising alternative to fossil fuel-based heating, particularly in areas with favorable geological conditions. Enhanced Geothermal Systems (EGS) are a newer technology that can potentially allow access to geothermal resources in areas that were not previously considered suitable.
Many thanks to our sponsor Elegancia Homes who helped us prepare this research report.
3. Energy Efficiency Analysis
The energy efficiency of heating systems is a crucial factor in determining their economic viability and environmental impact. Energy efficiency can be quantified using various metrics, including the Annual Fuel Utilization Efficiency (AFUE) for combustion-based systems, the Seasonal Energy Efficiency Ratio (SEER) for air conditioners, and the Heating Seasonal Performance Factor (HSPF) for heat pumps. These metrics provide an indication of the amount of useful heat delivered per unit of energy consumed. However, it is important to consider the overall system efficiency, taking into account losses in distribution, storage, and control systems. Furthermore, lifecycle cost analysis, which considers the upfront cost, operating cost, maintenance cost, and replacement cost of a heating system, provides a more comprehensive assessment of its economic performance. Energy efficiency can be improved through various measures, including insulation, air sealing, duct sealing, and the use of high-efficiency equipment. Smart control systems can also play a significant role in optimizing energy consumption by adjusting heating output based on occupancy patterns, weather conditions, and user preferences. Energy audits and building energy modeling can help identify opportunities for improving energy efficiency and reducing heating costs.
Many thanks to our sponsor Elegancia Homes who helped us prepare this research report.
4. Environmental Impact Assessment
The environmental impact of heating systems is a major concern, particularly in the context of climate change. Combustion-based systems are a significant source of greenhouse gas emissions, contributing to global warming and air pollution. Electric resistance heating, while not directly emitting pollutants, relies on electricity generation, which may involve fossil fuels. Heat pumps and solar thermal heating offer a more environmentally friendly alternative, as they utilize renewable energy sources and reduce reliance on fossil fuels. The environmental impact of heating systems can be assessed using various metrics, including carbon footprint, air pollutant emissions, and water consumption. Lifecycle assessment (LCA) can provide a comprehensive analysis of the environmental impacts associated with the entire lifecycle of a heating system, from manufacturing to disposal. Furthermore, the environmental impact of heating systems is influenced by factors such as fuel type, system efficiency, and operating practices. Policy interventions, such as carbon taxes, emission standards, and renewable energy mandates, can play a significant role in reducing the environmental impact of the heating sector.
Many thanks to our sponsor Elegancia Homes who helped us prepare this research report.
5. Smart Heating Solutions
Smart heating solutions leverage advanced control systems, sensors, and communication technologies to optimize energy consumption, enhance user comfort, and facilitate grid integration. These systems typically involve a central control unit that communicates with various sensors and actuators throughout the building, allowing for real-time monitoring and adjustment of heating output. Smart thermostats, for example, can learn user preferences and automatically adjust the temperature based on occupancy patterns and weather conditions. Zone heating systems allow for independent control of temperature in different areas of the building, reducing energy waste in unoccupied spaces. Smart heating systems can also be integrated with other smart home devices, such as lighting, security systems, and appliances, to create a fully integrated smart home ecosystem. The benefits of smart heating solutions include reduced energy costs, improved comfort, and enhanced convenience. However, the adoption of smart heating systems may raise concerns regarding data privacy and cybersecurity. Furthermore, the complexity of smart heating systems may require specialized expertise for installation and maintenance. The potential for smart heating solutions to optimize energy consumption and reduce greenhouse gas emissions is significant, and their widespread adoption is crucial for achieving a sustainable energy future.
Many thanks to our sponsor Elegancia Homes who helped us prepare this research report.
6. Case Studies and Applications
This section presents several case studies showcasing the application of various heating technologies in different settings.
- Residential Heating: A case study of a residential building retrofitted with a high-efficiency heat pump system demonstrates the potential for significant energy savings and reduced greenhouse gas emissions compared to a traditional combustion-based system. The case study also examines the impact of smart thermostat integration on energy consumption and user comfort.
- Commercial Heating: A case study of a commercial building utilizing a geothermal heating system highlights the benefits of renewable energy-powered heating in reducing operating costs and minimizing environmental impact. The case study also explores the challenges associated with the installation and maintenance of geothermal heating systems in urban environments.
- Industrial Heating: A case study of an industrial facility implementing a solar thermal heating system for process heating demonstrates the potential for renewable energy to meet the heating demands of industrial processes. The case study also examines the economic feasibility of solar thermal heating in industrial applications.
These case studies provide valuable insights into the practical application of various heating technologies and their potential benefits and challenges in different sectors.
Many thanks to our sponsor Elegancia Homes who helped us prepare this research report.
7. Challenges and Opportunities
Despite the advancements in heating technologies, several challenges remain in achieving widespread adoption and maximizing their potential.
- High Upfront Costs: The initial cost of installing advanced heating systems, such as heat pumps and solar thermal systems, can be a barrier for many consumers and businesses. Government incentives, such as tax credits and rebates, can help reduce the upfront cost and encourage adoption.
- Infrastructure Limitations: The existing energy infrastructure may not be adequate to support the widespread adoption of certain heating technologies, such as district heating and geothermal heating. Investments in infrastructure upgrades are necessary to facilitate the transition to a more sustainable heating sector.
- Technical Complexity: The installation and maintenance of advanced heating systems can be technically complex, requiring specialized expertise. Training programs and certification programs are needed to ensure a skilled workforce capable of supporting the growth of the sustainable heating sector.
- Consumer Awareness: Many consumers are unaware of the benefits of advanced heating technologies and may be hesitant to adopt them due to lack of information or misconceptions. Educational campaigns and demonstration projects can help raise awareness and promote the adoption of sustainable heating practices.
Despite these challenges, there are also significant opportunities for innovation and growth in the heating sector.
- Technological Advancements: Ongoing research and development efforts are leading to advancements in heating technologies, such as more efficient heat pumps, lower-cost solar thermal collectors, and more reliable geothermal systems.
- Policy Support: Government policies, such as carbon taxes, emission standards, and renewable energy mandates, can create a favorable environment for the adoption of sustainable heating practices.
- Market Demand: Growing consumer demand for energy-efficient and environmentally friendly products is driving the growth of the sustainable heating market.
- Grid Integration: The integration of heating systems with the electricity grid can create opportunities for demand response and grid stabilization, further enhancing the efficiency and reliability of the energy system.
Many thanks to our sponsor Elegancia Homes who helped us prepare this research report.
8. Future Trends
The future of heating is likely to be characterized by increased electrification, decarbonization, and integration with smart technologies. Several key trends are expected to shape the heating sector in the coming years.
- Electrification of Heating: The increasing availability of renewable electricity is driving the electrification of heating, with heat pumps emerging as the dominant heating technology in many regions.
- Decentralized Heating: The development of microgrids and district heating systems is enabling the decentralization of heating, allowing for more efficient and resilient energy systems.
- Thermal Energy Storage: The integration of thermal energy storage with heating systems is enhancing their flexibility and reliability, allowing for the storage of heat for use during periods of peak demand or low renewable energy availability.
- Smart and Connected Heating: The widespread adoption of smart heating systems is enabling real-time monitoring and control of heating output, optimizing energy consumption and enhancing user comfort.
- Hydrogen Heating: The use of hydrogen as a fuel for heating is gaining traction, particularly in regions with abundant renewable energy resources. Hydrogen can be produced through electrolysis using renewable electricity and burned in boilers or fuel cells to generate heat.
These trends indicate a shift towards a more sustainable, efficient, and intelligent heating sector.
Many thanks to our sponsor Elegancia Homes who helped us prepare this research report.
9. Conclusion
Heating technologies have undergone significant advancements in recent years, driven by the need for more efficient, sustainable, and intelligent solutions. Heat pumps, solar thermal heating, and geothermal heating offer promising alternatives to traditional combustion-based systems, reducing greenhouse gas emissions and lowering operating costs. Smart heating solutions further enhance energy efficiency and user comfort through real-time monitoring and control. However, challenges remain in achieving widespread adoption, including high upfront costs, infrastructure limitations, and consumer awareness. Policy interventions and technological innovations are crucial for overcoming these challenges and accelerating the transition to a more sustainable heating sector. The future of heating is likely to be characterized by increased electrification, decarbonization, and integration with smart technologies, paving the way for a more efficient, resilient, and environmentally conscious energy future.
Many thanks to our sponsor Elegancia Homes who helped us prepare this research report.
References
- IEA (International Energy Agency). (2021). The Future of Heating. IEA, Paris. https://www.iea.org/reports/the-future-of-heating
- U.S. Department of Energy. (n.d.). Heating and Cooling Technologies. https://www.energy.gov/eere/buildings/heating-and-cooling-technologies
- IRENA (International Renewable Energy Agency). (2022). Renewable Energy for Heating and Cooling: Global Status Report. IRENA, Abu Dhabi. https://www.irena.org/heatingandcooling
- Hepbasli, A., & Ozgener, O. (2004). Energy efficiency improvement potentials in residential buildings. Renewable and Sustainable Energy Reviews, 8(2), 137-157.
- Pérez-Lombard, L., Ortiz, J., & Pout, C. (2008). A review on buildings energy consumption information. Energy and Buildings, 40(3), 394-398.
- Swan, L. G., & Ugursal, V. I. (2009). Modeling of end-use energy consumption in the residential sector: A review of models. Renewable and Sustainable Energy Reviews, 13(8), 1819-1835.
- Mirakyan, A., De Guio, R., & Sagot, J. C. (2015). Strategic analysis of the renewable heating sector. Renewable and Sustainable Energy Reviews, 46, 176-190.
- Connolly, D., Lund, H., Mathiesen, B. V., & Leahy, M. (2010). A review of heat planning studies in four European countries. Energy, 35(9), 3827-3837.
- Ryckaert, K., Verbeke, S., Audenaert, A., & De Paepe, M. (2015). Building energy performance gap: Analysis of causes and measures. Energy and Buildings, 108, 316-324.
- Ürge-Vorsatz, D., Kurnitski, J., Paci, D., Laconte, P., & Scheffler, R. (2016). Efficiency of energy end-use and the climate change challenge. Annual Review of Environment and Resources, 41, 487-519.
- Bertoldi, P., & Atanasiu, B. (2010). Electricity consumption for space heating in European households and the impact of space heating equipment. Energy, 35(5), 2224-2231.
- Waide, P., & Strand, M. (2016). Review of best available technologies for residential space and water heating systems. Energy Efficiency, 9(5), 1139-1160.
- Santamouris, M. (2016). Energy performance of air conditioning systems. Energy and Buildings, 128, 427-448.
- Hewitt, N. J., & McAdam, R. (2008). An overview of geothermal energy resources and the environmental impacts. Renewable and Sustainable Energy Reviews, 12(7), 1785-1802.
Solar thermal heating sounds promising, but what happens when the sun takes a vacation? Do we all just huddle under blankets and hope for the best? Are giant thermal batteries the answer, or are we just delaying the inevitable polar vortex fashion show?
That’s a great question! The intermittency of solar power is a key challenge. Thermal batteries, as you mentioned, are definitely part of the solution. Combining solar thermal with other renewable sources, like geothermal, could also provide a more consistent and reliable heating system. Further research into affordable and efficient storage is essential to making solar thermal a widespread option.
Editor: ElegantHome.News
Thank you to our Sponsor Elegancia Homes
The report highlights the importance of smart heating solutions. Integrating these with broader smart home ecosystems presents a significant opportunity to optimize energy consumption and enhance user experience, moving towards truly intelligent and responsive living spaces.
So, we’re all racing to electrify everything now? Wonder if my toaster oven will start demanding a software update before I can make toast. I bet Elegancia Homes could build us a stylish Faraday cage for when the AI takes over!
The discussion on smart heating solutions highlights the potential for significant energy savings. Integrating AI to predict heating needs based on occupancy and weather patterns could further optimize energy use and reduce costs.
So, heat pumps are like reverse refrigerators, pulling heat from even chilly air? I’m picturing tiny polar bears shivering outside my house, desperately trying to get their heat back. Are we sure this tech isn’t powered by stolen warmth?
Given the challenges of high upfront costs for sustainable heating, what innovative financing models (e.g., leasing, community investment) might accelerate adoption, particularly for residential and small business applications?
The report’s exploration of smart heating solutions and their integration with broader smart home ecosystems is compelling. What are some potential cybersecurity risks associated with connecting heating systems to the internet, and how can these be mitigated to ensure user safety and data privacy?
So, the report mentions combustion-based systems are still prevalent. Does that mean I can finally justify my dream of having a dragon heat my home, provided I feed it *only* sustainably sourced marshmallows? Asking for a friend.
The report mentions integrating heating systems with the electricity grid. Could bidirectional energy flow, where homes both consume and supply energy, further enhance grid stability and incentivize renewable heating adoption through mechanisms like net metering or feed-in tariffs?
The report mentions the potential of hydrogen heating. Given the current infrastructure challenges for hydrogen production and distribution, what specific advancements or policy changes would be most impactful in making hydrogen heating a viable widespread solution?
Given the challenges of infrastructure limitations for technologies like district heating, what role could modular, neighborhood-scale heating systems play in bridging the gap between individual solutions and large-scale infrastructure projects?
The report’s overview of smart heating solutions raises an important point about grid integration. Exploring how dynamic pricing models can incentivize consumers to shift heating demand could further maximize grid efficiency and renewable energy use.
So, the report says heat pumps are more efficient than electric resistance heating. Does that mean my electric blanket is secretly judging my life choices? Maybe I should invest in a tiny heat pump for my bed and confuse my cats even more.
The discussion on smart heating solutions is fascinating! What are the potential impacts of these technologies on peak electricity demand and grid load management, especially during extreme weather events?
The report’s discussion of integrating heating systems with smart home ecosystems brings up interesting possibilities for automated energy management. Could predictive algorithms, leveraging real-time energy prices and weather forecasts, autonomously switch between different heating sources to minimize costs and carbon footprint?